Quantum Computing
Prime Movers Lab
May 2022
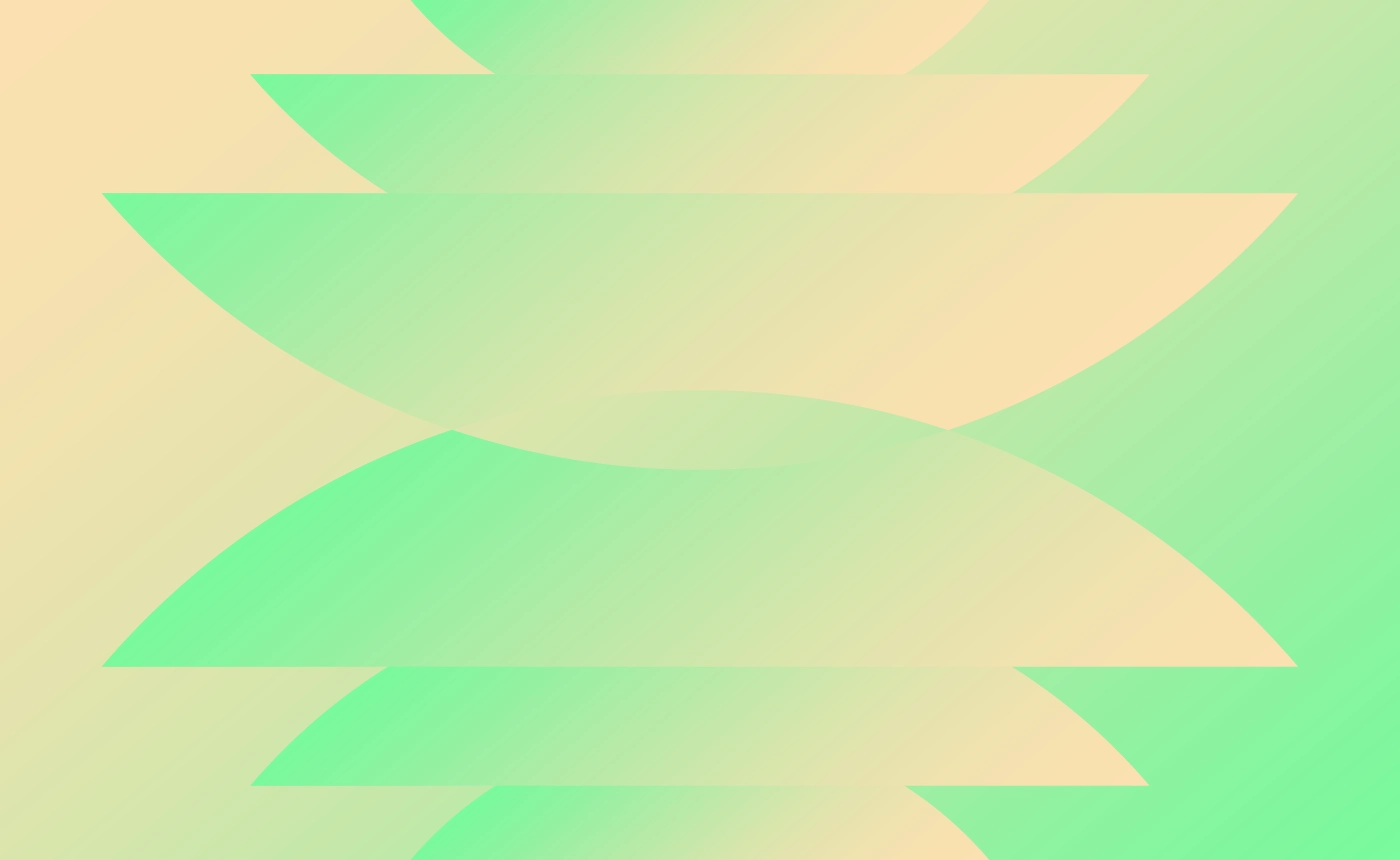
Executive Summary
Quantum computing is a technology that could transform billions of lives by enabling humans to solve previously intractable problems in optimization, chemistry, advanced materials, and other areas. However, it still has a long way to go to realize these promises.
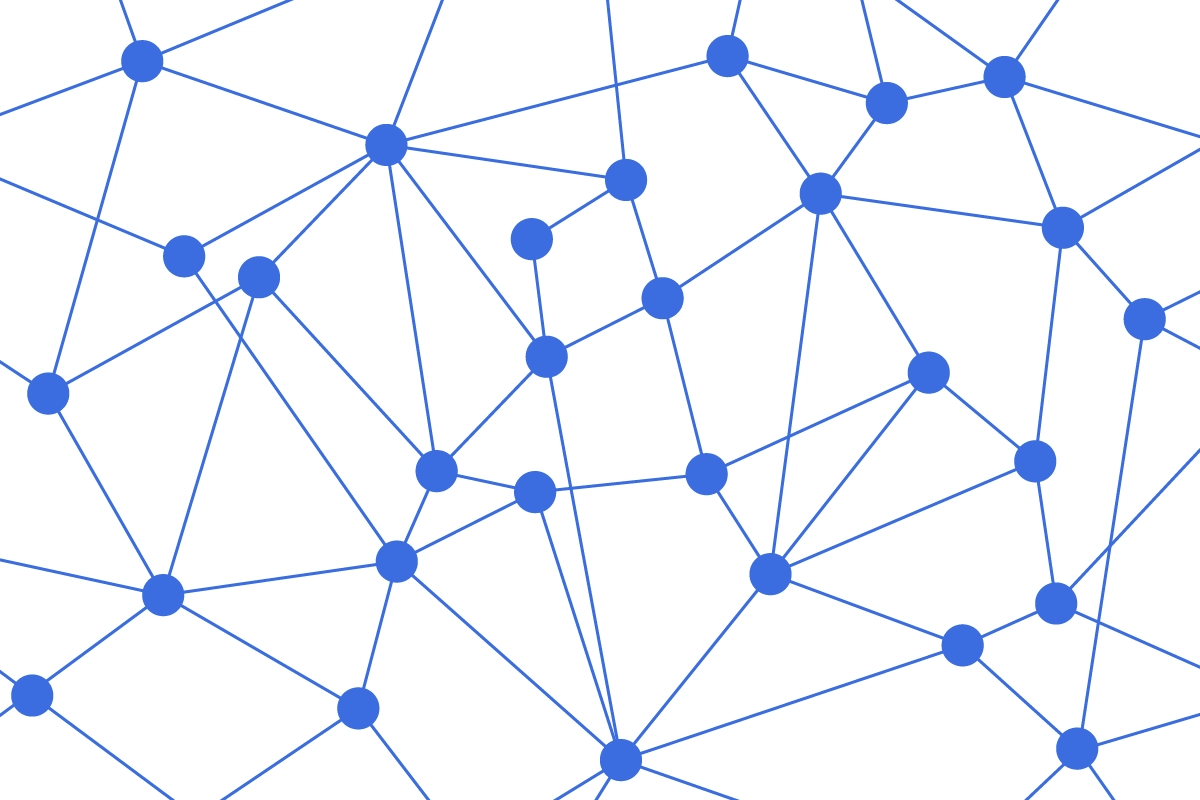
Figure 1. Quantum computing promises to solve previously impossible problems in fields like optimization.
Macro Trends: Reduced Cost of Launch AND Moore’s Law
Key takeaways on quantum computing companies in general:
- The number of quantum computer companies is small compared to other sectors we have evaluated, like alternative proteins, batteries, or satellites. Many of the groups listed are university or government labs, or departments within larger tech companies (Google, IBM, Amazon)
- Superconducting qubit-based approaches are the most researched (and have received the most development resources)
- Almost all of the startups in this space are based on technology from university labs
- Due to the intense specialization required and limited talent pool for quantum technology development, the competition for talent in the quantum computing space is fierce
Quantum Computing is a relatively new field of interest for investors. A wave of startups was founded around 2017-2018. Three years later, the total dollar amount invested has increased from less than $500 million to roughly $3 billion as companies like DWave, PSIQuantum, and Xanadu have raised large, later-stage rounds. Investor activity before 2021 was almost entirely venture capital. The first significant exit in this space was quantum encryption company ArQit (NAS: ARQQ) which went public via SPAC for $1B in 2021. Then, IonQ (which is developing quantum computers using trapped ions as qubits) followed, going public via SPAC at a $2B valuation. ). In October 2021, Rigetti (superconducting qubits) announced the approval of its SPAC at a $1.5B valuation.
Why invest in quantum computing? There is clearly a momentum play here - several companies have shown an ability to exit, albeit via SPAC and for relatively small numbers, modest returns. Critically, we believe that a market for quantum computing as a cloud service will materialize. These services may become a must-have for finance, help solve important logistics problems, and become a standard tool for pharma and advanced material companies. In 2020, Amazon Web Services (AWS) generated $45.4B in revenues from its cloud services division (30 percent year growth). Quantum computing companies like IQM and Rigetti are already generating revenues from their cloud offerings. If some fraction of all cloud services is handled by quantum computers in the future, quantum computing providers would generate significant revenues.
What are we looking for in a quantum computing company?
- Team: The team has a track record of hitting technical milestones, publishes research and findings to establish credibility with the scientific community, and can recruit strong talent. The team has connections to future customers and/or acquirers.
- Tech: We are looking for a technology that can be scaled to many logical qubits in a capital-efficient way, and be applied to a wide range of problems (approaching a universal quantum computer). Science risk is eliminated; the remaining technical risk is mostly engineering, i.e. there is a clear path to achieving the remaining technical milestones that don’t require miracles.
- Business: We want to see early engagement with future customers (companies like Amazon, pharma, finance, defense), and that the company will be able to tell a story about going public in the not-so-distant future.
Technology
A brief introduction
Remember the first computer? Probably not.
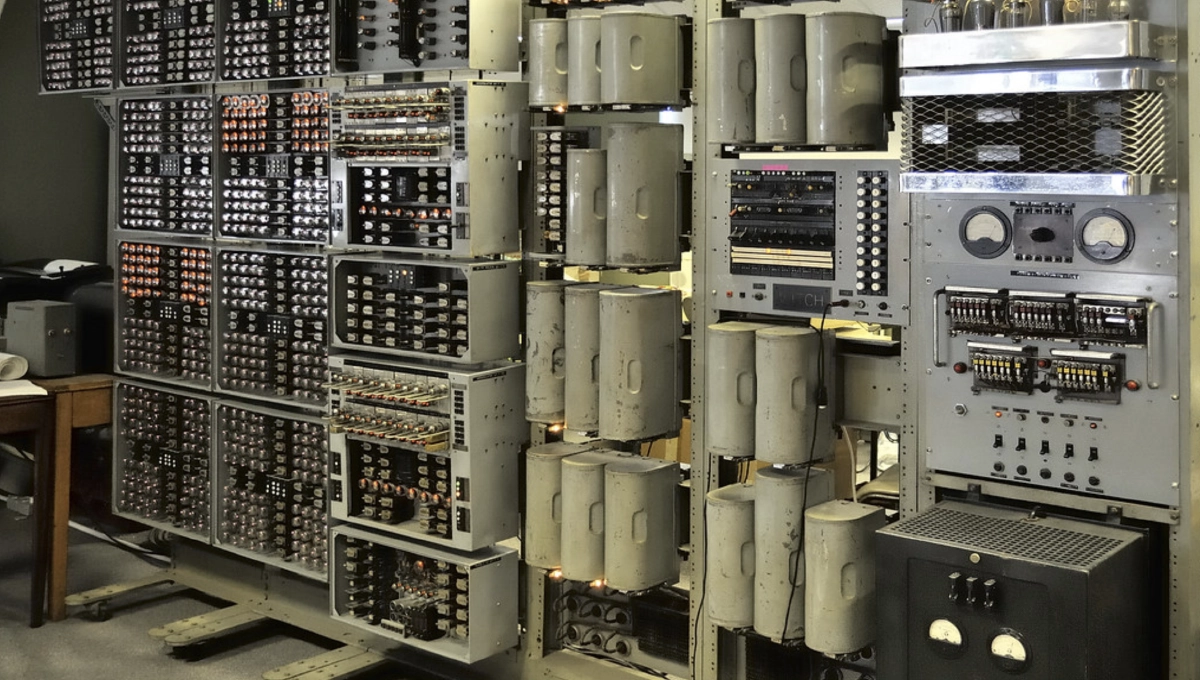
Figure 2. Harwell Dekatron vacuum-tube (thermionic valve) computer. Source: EDK7
The on/off variations in electrical flow reflects the number of valves present in a computer system. These valves, also known as vacuum tubes, conduct and alter the flow of electricity, providing the necessary on/off signals. Valve computers were massive in size, sometimes filling a whole room, and they created a lot of heat, putting them at risk of burnout.
Fast-forwarding 70 years, computers are built with circuits using tiny transistor components, enabling them to be both extremely powerful and miniaturized. But our ability to squeeze more transistors onto a silicon-based chip (the famous Moore’s Law) is nearing an end due to physical limitations. Researchers and companies are working on the next-generation technology to create more powerful computers that bring humanity past the silicon transistor era. A category of computers that solves problems in a fundamentally different way is Quantum computing: research, governments, and private companies are leading the way to bring this technology to fruition.
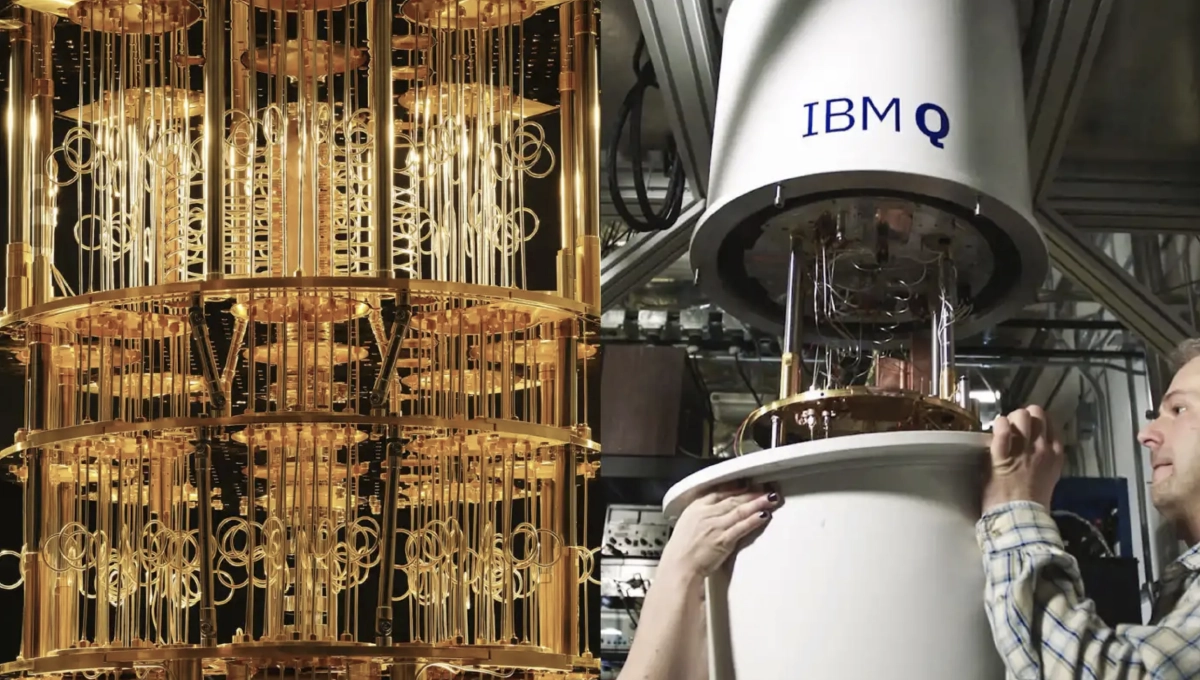
Figure 3. IBM Quantum System One. Photo courtesy of IBM.
The concept of quantum computers has existed since the 1970s. In the 1990s, researchers were comfortable enough with the concept of quantum computing to begin constructing creative algorithms (methods of solving problems) on it, assuming it existed. In 1994, Peter Shor developed his renowned method detailing how quantum computers might factor huge numbers while cracking RSA encryption. It's safe to assume that math came first, followed by engineering.
A UC Berkeley/MIT/Los Alamos collaboration created the first quantum computer (with just two qubits) in 1998. Shor's algorithm was initially demonstrated on a quantum computer in 2001 by scientists and Stanford graduate students at IBM's Almaden Research Center in San Jose. These first quantum computers were little more than chemicals in a test tube.
Quantum computer research and development has advanced dramatically since 2000. The discovery of new approaches to control the quantum states of atoms, electrons, and photons has increased the number of choices for constructing quantum computers. And the number of qubits achieved, as well as the number of businesses involved in quantum computing technology, has grown.
What is a Quantum computer?
A quantum computer is a system that performs calculations using qubits rather than bits. Bits are used as data units in traditional computers (zeros and ones). Quantum computers employ qubits, which may represent both zero and one at the same time due to a quantum mechanics phenomenon known as superposition.
Quantum mechanics is the part of physics that looks at subatomic particles (the physics of things smaller than atoms): electrons, light, radio waves, and other forms of energy. For things bigger than atoms, and at speeds much lower than the speed of light, “classical” physics works just fine. When you get smaller or faster, classical physics falls apart — you need quantum mechanics to accurately describe things.
At the quantum scale, three unique properties emerge: superposition, entanglement, and tunneling. These features allow quantum computers to be exponentially faster than today's mainframes and servers at solving some classes of problems. It also means that quantum computers should more accurately model physical, real-world systems at the atom scale - important for materials science and drug discovery, for example. Traditional computers can handle only a set of inputs and one calculation at a time. Quantum computers, using for example n qubits, can calculate 2n inputs at once.
What is a qubit?
Quantum computers use qubits, which are quantum bits supporting on, off, and in-between states.
Superposition
Qubits can represent a combination of both zero and one at the same time, based on a principle called superposition. Superposition is a state of a qubit where it is simultaneously on and off (like 30 percent zero and 70 percent one, for example). The superposition principle asserts that something – an electron, an atom, a photon — exists in all conceivable states simultaneously until it is measured. A famous example is the “Schrödinger's cat” experiment. As explained on Wikipedia, “Schrödinger's cat: a cat, a flask of poison, and a radioactive source are placed in a sealed box. If an internal monitor (e.g. Geiger counter) detects radioactivity (i.e. a single atom decaying), the flask is shattered, releasing the poison, which kills the cat. The Copenhagen interpretation of quantum mechanics implies that after a while, the cat is simultaneously alive and dead. Yet, when one looks in the box, one sees the cat either alive or dead, not both alive and dead. This poses the question of when exactly quantum superposition ends and reality resolves into one possibility or the other.”
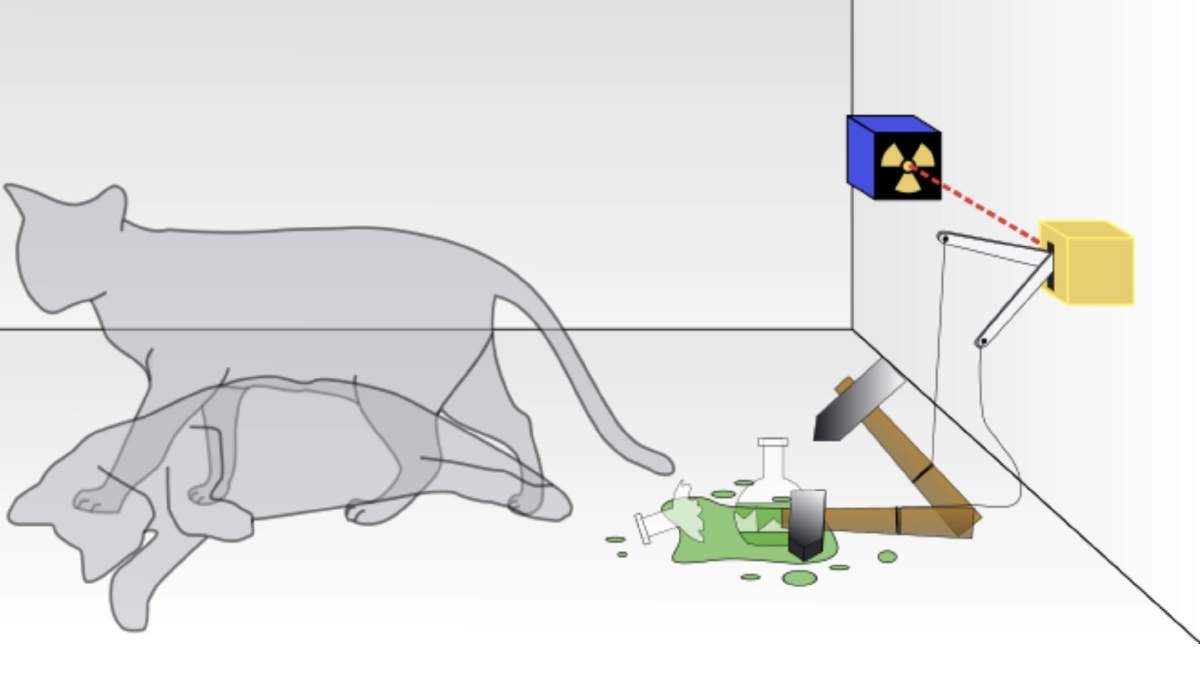
Figure. 4. Schrödinger's cat experiment. Source: Wikipedia, Dhatfield under CC
Superposition, in practice, allows future quantum computers to tackle problems that are too complicated for conventional (classical) computers by examining several potential configurations at once. In a nutshell, quantum computing is a sophisticated approach to making parallel calculations, using the physics that governs subatomic particles to replace the more simplistic transistors in today’s computers, as stated in this article.
Entanglement
Two or more qubits whose quantum states are coupled (they share the same wavefunction) are called entanglement. Quantum entanglement is a quantum mechanical phenomenon in which the quantum states of two or more objects have to be described with reference to each other, even though the individual objects may be spatially separated. This results in correlations between the systems' observable physical characteristics. For example, it is feasible to create two particles in a single quantum wave function so that when one is observed to be spin-up, the other is always observed to be spin-down and vice versa, despite the fact that quantum physics makes it impossible to anticipate which set of measurements will be observed.
As a result, measurements made on one system appear to have an instantaneous impact on other systems that are entangled with it.
Changing the state of an entangled qubit instantaneously changes the state of the paired qubit in quantum computers. As a result, entanglement accelerates the processing speed of quantum computers. Because processing one qubit reveals information about many qubits, doubling the number of qubits does not necessarily increase the number of processes (i.e. the entangled qubits).
Quantum Supremacy
The definition of quantum computing supremacy — the point at which quantum computers can do jobs that conventional computers cannot — is a hotly debated topic in the industry.
Quantum Gates
In order to process the qubits, as in classical computing, a quantum circuit applies gates (using for example microwaves or lasers) to manipulate the quantum information and finally compute an answer. There is one other technique, called quantum annealing which is used by the company D-Wave (one of the industry leaders). Quantum annealing is a metaheuristic for finding the global minimum of a given objective function over a given set of candidate solutions (candidate states), by a process using quantum fluctuations. It works well on very specific problems like optimization and, if you were interested, you can pick up a quantum annealing computer from D-Wave for $10-15 million.
Quantum Volume
Quantum volume is a metric that considers the number of qubits, connectivity, and gate fidelity. The quantum’s volume numerical value is representative of the complexity of the specific problem solved by a quantum computer. The width and depth of a quantum circuit refer to the number of qubits and operations that may be done. The more sophisticated an algorithm the computer can perform, the deeper the circuit. The number of qubits, how qubits are coupled, gate and measurement errors, device cross-talk, circuit compiler efficiency, and other factors, all impact circuit depth.
Challenges with Quantum Computing
There are several challenges, with the most significant being fault tolerance. Qubits are extremely difficult to maintain. Even the tiniest amount of noise (background radiation, electromagnetism, or a big sneeze) affects the qubit, rendering it meaningless. Currently, it is believed that 1,000,000 physical qubits will contain only 1,000 usable qubits when error correction using the redundancy principle is applied. Increasing the stability of qubits is paramount for creating a Quantum Universal Computer capable of handling any algorithm — a goal that is realistically yet 10-15 years away.
Quantum Computing Outlook
IBM, Honeywell, IonQ, DWave, Xanadu, and Google have early versions of quantum computers. Today, they have tens of qubits. Unfortunately, today qubits are noisy, so tens of hundreds of thousands of qubits are needed to successfully solve real-world problems.
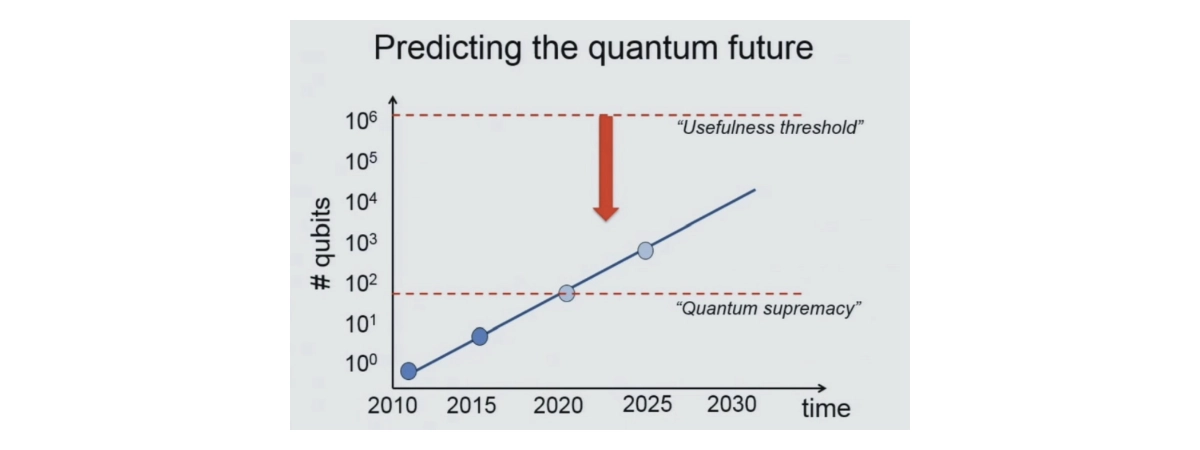
Figure 5. Estimated growth of qubits. Courtesy of NVIDIA
There is no consensus, but the prediction for a useful quantum computer is to have 10k-1M qubits.
Quantum Computing Technologies
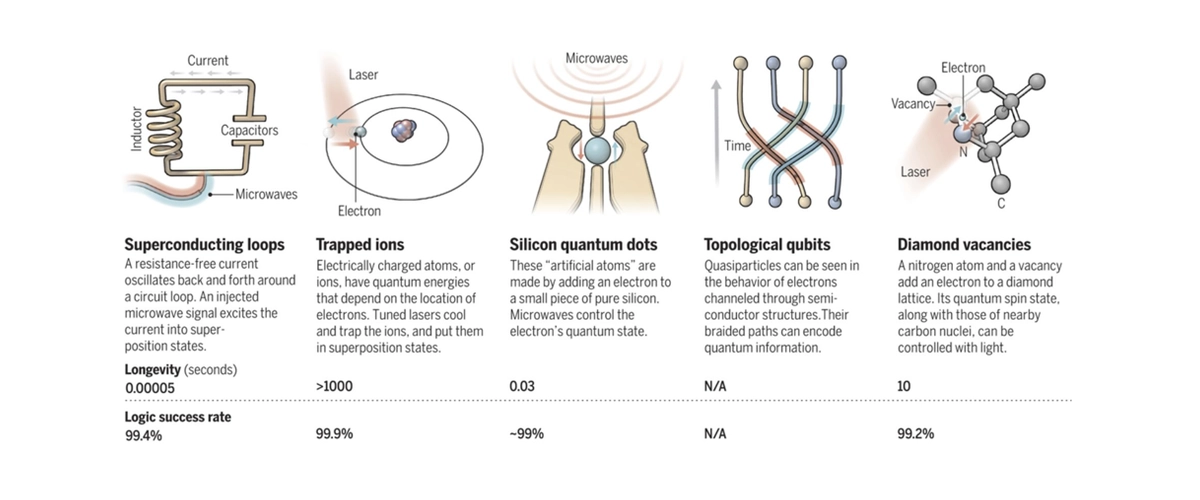
Figure 6. Different technologies for qubits. Source: C. Bickle/science data by Gabriel Popkin
Different qubits can be utilized to solve different challenges, depending on the application. For example, photons are good for quantum communication since they are the only qubits that can travel and be kept at room temperature indefinitely.
Most IT giants – including Google, IBM, Intel – exploit the quantum characteristics of electrons in superconducting circuits to create quantum computers. That approach is also developed by large startups like Rigetti and IQM. Cryogenic temperatures (about 15 milli-Kelvin) are required for this. Superconducting qubits are the most researched and resourced approach to building quantum computers. These qubits consist of Josephson Junctions: a pair of superconducting metal strips separated by a tiny nanometer-wide gap that electrons cross, leading to quantum effects. The superconducting qubits are manipulated with microwave pulses (SPIE 2020). These quantum computers require huge dilution refrigerators (very different from your fridge at home!) to keep the qubits near absolute zero.
Microsoft is developing topological quantum computing. These more exotic “topological” qubits don’t store the information by changing the energy of particles but rather by affecting how the particles interact with each other — their topology. Topological qubits are, in theory, more stable than qubits encoded in individual particles but are also farther from commercialization. In topological quantum computers, the qubits are anyons (a little-known quasiparticle). Observing these quasiparticles was a huge physics breakthrough, and won the 2016 Nobel Prize in Physics (Washington Post explainer). Topological qubits also require the chips to be held at low temperatures.
D-Wave, an industry leader, is developing quantum annealing computing. Quantum annealing is a metaheuristic for finding the global minimum of a given objective function over a given set of candidate solutions (candidate states), by a process using quantum fluctuations. It works well on very specific problems like optimization.
Companies like IonQ and the conglomerate Honeywell are leading the charge in trapped-ion quantum computing, which involves trapping ions within a vacuum. Ions (charged atoms or protons), can be confined and suspended in free space using electromagnetic fields. Qubits are stored in stable electronic states of each ion, and quantum information can be transferred through the collective quantized motion of the ions in a shared trap (interacting through the Coulomb force). Lasers induce coupling between the qubit states (for single qubit operations) or coupling between the internal qubit states and the external motional states (for entanglement between qubits).
Atom Computing and Cold Quanta are using neutral atoms as qubits. Like ion-trap systems, qubits can be programmed using the energy levels of the atoms. Light, or electromagnetic radiation, can be used to trap and manipulate the quantum states of uncharged (neutral) atoms. Multiple qubits that are nearby in space can be programmed to interact with one another via two-qubit gates. This opens up some tantalizing possibilities for exotic quantum-computing circuit topologies.
The main hardware components constituting a neutral atom quantum processor are shown in Figure 7. The trapping laser light (in red) is shaped by the spatial light modulator (SLM) to produce multiple microtraps at the focal plane of the lens (see inset). The moving tweezers (in purple), dedicated to rearranging the atoms in the register, are controlled by a 2D acousto-optic laser beam deflector (AOD) and superimposed on the main trapping beam with a polarizing beam-splitter (PBS). The fluorescence light (in green) emitted by the atoms is split from the trapping laser light by a dichroic mirror and collected onto a camera. (b) Photography of the heart of a neutral-atom quantum co-processor. The register is prepared at the center of this setup.
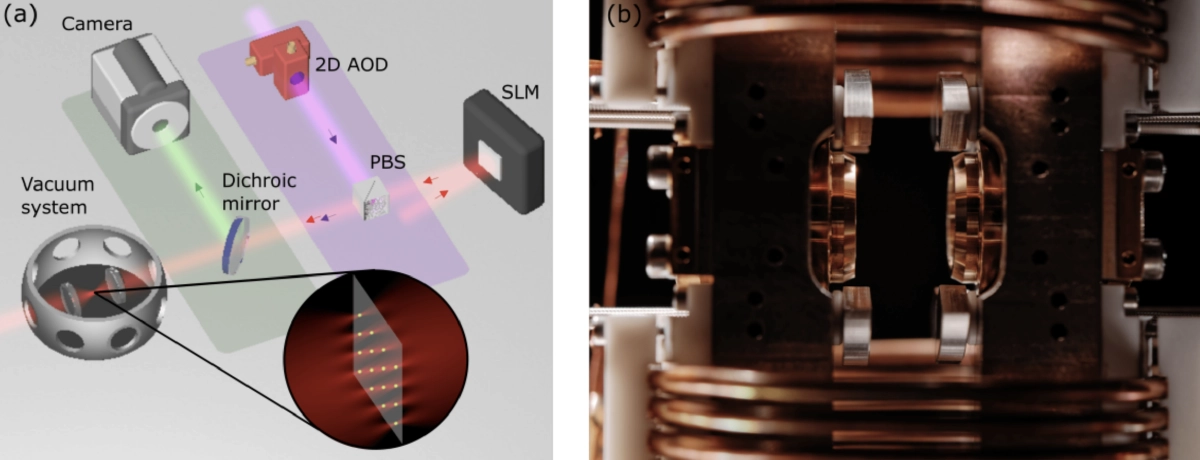
Figure 7. Overview of the main hardware components of a neutral atom quantum processor. Source: arXiv:2006.12326 [quant-ph]
Neutral atom platforms for quantum processing have a unique potential for scalability: the size of the quantum register is only limited by the amount of trapping laser power and by the performance of the optical system generating the optical tweezers.
Quantum computers based on silicon photonics use single photons in silicon photonic waveguides (i.e. pipes for photons) and are then manipulated using networks of optical components. PsiQuantum and Xanadu are working on this approach.
The startup EeroQ uses electrons on the surface of liquid helium as qubits.
Other approaches are still in development, including using nitrogen vacancy (NV) centers in diamonds as qubits, and silicon quantum dots.
General Quantum Computer Metrics
- The total number of (physical) qubits demonstrated: less than 100. The current record is from China with 76 qubits. There is confusion around the D-Wave claim and its 5k qubit machines. Larger quantum machines tend to have lower connectivity, which means that the qubits can’t communicate with one another quite as well. This lack of connectivity lowers the system’s overall computing power.
- How many qubits are needed for a useful system? 10k-1M (estimated).
- Number of qubit gates: A quantum gate is a basic quantum circuit operating on a small number of qubits. They are the building blocks of quantum circuits, like classical logic gates are for conventional digital circuits.
- Coherence time: How long can the qubits store information? Keeping the qubits in their states long enough to do a calculation is hard. This property is called coherence. Several factors can disturb a quantum state: temperature fluctuations, vibrations, etc. Quantum computer designers have to go to great lengths to isolate the qubits from the rest of the world.
- Error rate: Quantum computers are error-prone machines. These computing errors are inherent in quantum systems and cannot be avoided in and of themselves. That's why a lot of money and expertise is being spent on quantum error detection and determining how to construct machines that can identify and repair their own faults. Although considerable progress has been achieved in this field, it is doubtful that quantum mistakes will ever be completely eliminated. Even with very precise quantum computers, classical computers will be required to validate the final output. Quantum computers need thousands of logical qubits to do meaningful calculations. If many of the quantum computer’s qubits are subject to error, or rapidly decohere and lose the information they stored, it may take more than 1,000 physical qubits to make a single logical qubit.
- Energy consumption: At the moment, quantum machines are not efficient from a power consumption point of view.
The DiVincenzo criteria are conditions necessary for constructing a quantum computer. They were proposed in 2000 by the theoretical physicist David P. DiVincenzo. The criteria prescribe a computer that would be able to efficiently simulate quantum systems, such as in solving the quantum many-body problem. (These criteria build on the ideas proposed by mathematician Yuri Manin in 1980, and physicist Richard Feynman in 1982.)
DiVincenzo Criteria can be helpful in evaluating a startup's quantum computing technology:
- A scalable physical system with well-characterized qubits.
- The ability to initialize the state of the qubits to a simple fiducial state. One simple way to initialize a qubit is to wait long enough for the qubit to relax to its energy ground state. In addition, controlling the qubit potential by the tuning knobs allows faster initialization mechanisms.
- Long relevant decoherence times.
- A “universal” set of quantum gates.
- A qubit-specific measurement capability.
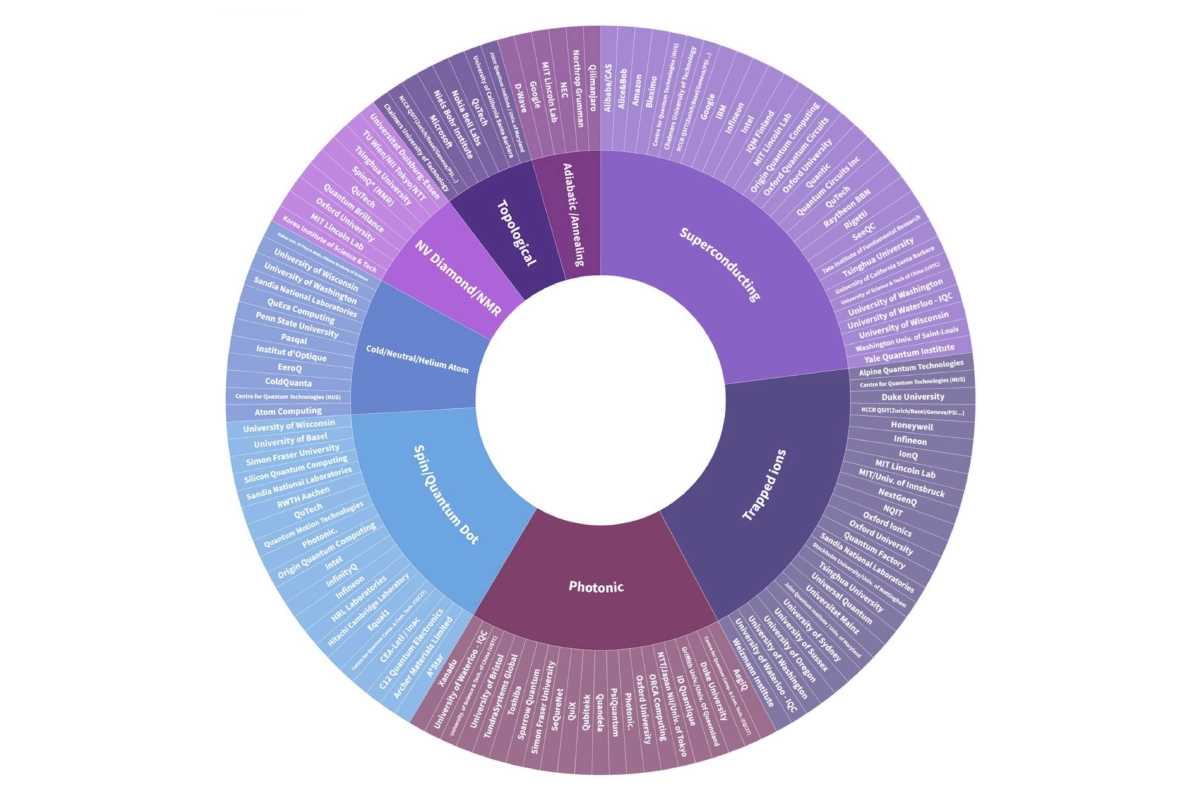
Figure 8. Different Quantum technologies with a map of the research and private sector players.
Source: Organisations Qubit technology, Michel Kurek
Applications
Quantum computer architecture is inherently different and has different strengths (and weaknesses) than classical computers, including:
- Memory: Classical computers can store information for long periods of time. By contrast, a quantum computer’s “memory” only lasts a few microseconds. Quantum memory is an active area of research for communication applications.
- Architecture/how much space the computer occupies: Most quantum computers require ultra-low temperatures close to absolute zero (-270 degrees Celsius or -450 degrees Fahrenheit) and/or advanced optics to run.
- Classical and quantum computers are good at solving different problems (algorithms).
The first useful (quantum advantaged) quantum computers will be hybrids with quantum processors running some operations, and classical processors running others. Having a hybrid also helps address the memory problem - intermediate results can be transferred to a classical memory chip. There are some areas where we know quantum computers will excel: simulating complex natural systems (chemicals, materials, weather), breaking RSA encryption, and specific algorithms. Quantum computers can encode much more complex information in a single quantum bit, due to superposition. This allows them to solve systems of complex equations (like the position and state of many electrons) more easily. It’s similar to the difference between measuring derivatives over and over to find the lowest point in a mountain range, and being able to see the entire landscape at once.
For quantum computer calculations, more qubits and computational time are required as the problem gets more difficult. The advantage is that the computation time scales only polynomially with the size of the problem, and not exponentially as for classical computers. Polynomial-time is much, much shorter than exponential time as n, the number of inputs or parameters to calculate (e.g. the number of electrons in a molecule to include) increases.
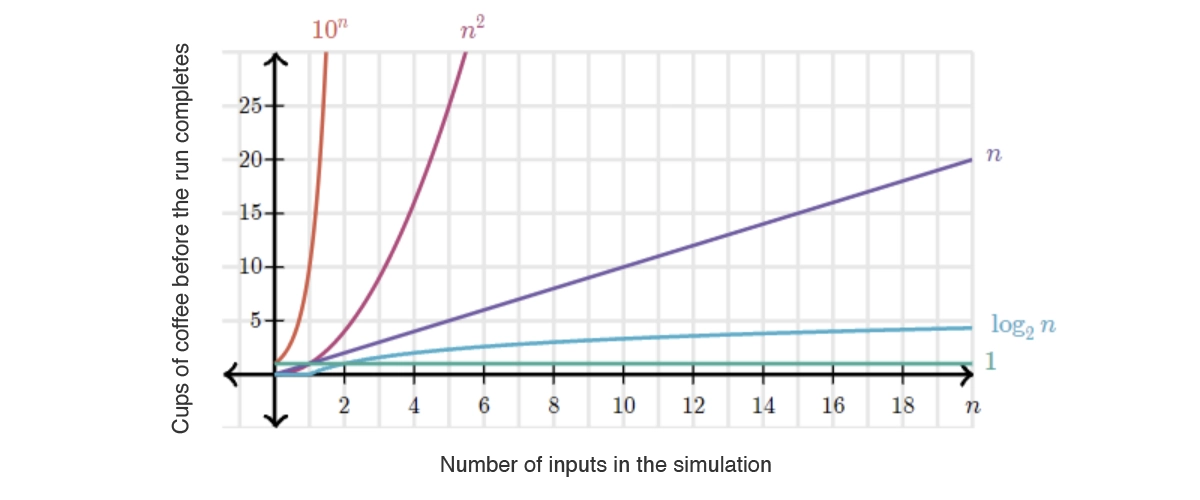
Figure 9. Example of exponential vs polynomial scaling. If the y-axis is how long your program takes to run, and n is the number of inputs to your model, you’ll want to use a method that scales polynomially (n or n2) rather than exponentially (10n).
Identifying problems that are classically hard and quantumly easy is a very active area of research, and this question is far from being definitively answered. It can be a challenging task to understand the potential business impact of quantum applications. This is one reason for the many collaborations between big corporations and research institutes or big tech players to understand and get “smart” in their strategy.
Two areas where quantum computing is expected to add value are finance and chemical and materials discovery. However, there are many other problems (specifically graph problems and optimization research) where quantum computers may excel, i.e. for scheduling, and resource optimization, like the traveling salesman problem.
Finance
In a data-heavy environment, ever-more-powerful computers are required to calculate probability properly. With this in mind, some banks are turning to a new breed of computers that use quantum physics principles to crunch massive quantities of data quickly. These institutions are able to evaluate big or unstructured data sets efficiently. Sharper insights into these categories may help banks make better decisions and provide better customer care, such as through more timely or relevant offerings (perhaps a mortgage based on browsing history). Capital markets, corporate finance, portfolio management, and encryption-related operations all have compelling application cases. This can be a path to true competitive advantage in an increasingly commoditized world. Quantum computers are especially interesting in applications where algorithms are driven by live data streams, such as real-time market prices, which include a high amount of random noise.
Financial and economic crises during the previous two decades have resulted in significant changes in how banks and other market players assessed and priced risk across asset classes. As a result, increasingly complicated and real-time risk models driven by artificial intelligence but still based on traditional computers were introduced.
When determining where quantum computing would be most beneficial, four capital markets sector archetypes should be considered: sellers, buyers, matchmakers (including trading platforms and brokers), and rule setters.
In general, sellers and matchmakers spend in information technology (IT) to increase capacity rather than solve complexity. Buyers and rule makers, on the other hand, frequently want more sophisticated models. For example, quant-driven hedge funds seek to profit on analytical complexity. As a result, they would be an obvious target for ultra-powerful processing. Large banks, which play various roles in financial markets, are also early adopters.
If you want to learn more about fintech and quantum, read this McKinsey article.
Out of the 160+ industry partners working with IBM’s quantum computers, seven are financial services firms, including JP Morgan, Barclays, Goldman Sachs, and Wells Fargo. Overall there are currently 20 financial institutions in the quantum ecosystem.
Goldman Sachs and Citigroup also invest directly in quantum computing companies like D-Wave and QC Ware.
Chemicals and Pharmaceuticals
Quantum computers are much better suited to simulate molecules and materials (and find better ones!) than “classical” computers because they follow quantum mechanics. It’s really hard to simulate chemicals with lots of electrons or possible configurations. Solving the equations to describe the location and movement of electrons on a classical computer takes a huge amount of processing power and memory. Even with today’s supercomputers, we can barely get exact solutions for simple molecules. For more science, see this thought piece on quantum chemistry.
Beware of the hype! Exact solutions (i.e. quantum computers) aren’t always needed to design new and better molecules. Quantum chemistry is a mature field – researchers routinely calculate important chemical properties using approximations, many of which are darn good. The pace of computer-assisted drug discovery has, in fact, been accelerating for decades without quantum computers. Example: Density functional theory (DFT) was a huge breakthrough in the 1990s that allowed chemists to make much more accurate chemical structure predictions.
Looking ahead, the continued increase in the computation power, distributed cloud-based computational resources, and the miracles of AI/ML are giving chemists and biochemists more powerful tools. Dozens of AI/ML startups are already on the hunt for better candidates for pharma leads, battery materials, and even low-carbon cement formulations. Many are producing good hits without using quantum computers.
Quantum computing can stand out where the approximations for classical computers break down. Examples include molecules containing metal atoms (which have many associated electrons), materials with large shared electron systems, and regions of a molecule/drug where super high accuracy is key.
Examples of important chemical and biological molecules that are difficult to simulate with classical systems are:
- A DNA regulation enzyme histone demethylase, which helps switch genes on/off
- The non-covalent binding between biotin and the avidin protein, which has been used for nanoscale drug delivery systems
- Biological nitrogen fixation by the enzyme nitrogenase, which converts nitrogen into two ammonia molecules under ambient conditions.
- The N-chromophore photosynthetic complex, which plants use to efficiently convert sunlight and CO2 into organic molecules.
- Materials showing high-temperature superconductivity, which would be useful for applications in energy and electronics.
- Super high accuracy is important in drug discovery studies, i.e. calculating the binding affinity of drug active regions in different liquids and protein environments.
To put things in perspective: the ability to use quantum computers to create new, improved molecules is only the first step in the process. Scientists then need to find a way to make the molecule correctly, stabilize it, scale-up production, ensure quality control, find distribution channels, and make sales. This is a long road.
Major pharma companies have expressed interest: Amgen, Biogen, Genentech, Pfizer and others have projects with quantum simulation companies (many of whom hope to harness quantum computers when they become available).
Meanwhile, large companies have quantum computing research divisions exploring how to use quantum computers to solve compelling (or not so compelling) problems:
- Microsoft’s team is working to understand how nitrogenase converts atmospheric nitrogen into fertilizer at ambient temperatures. (Journal article)
- IBM’s team is calculating dissociation curves for simple molecules found in lithium sulfur batteries. (Journal article)
- Google wants to be acknowledged for reaching quantum supremacy. They also made ‘Time Crystals’.
- An interesting use case is how IBM quantum computing services were used by Daimler to simulate the chemical reactions, at a molecular level, in lithium batteries. Simulating how the electrons react with the molecule takes days and it’s just an approximation. Simulating the full chemical reaction is too complicated. This is a specific use case driven by the macrotrend of being net-carbon zero by 2030 and Daimler shifting to full electric technology. Read the full case study here.
Business Models
In most cases, quantum computing will be primarily offered as a cloud service (Quantum Computing as a Service, or QCaaS). In the last few years, there’s been a race to provide public cloud-based quantum services. For example:
- Microsoft has been building quantum computing services for over a decade, including a quantum computing service in Azure that can be accessed remotely by users. The service currently simulates 30–40 qubits.
- IBM launched its “Q Network” in 2016 to provide access to the company’s experts, developer tools, and cloud-based quantum systems for organizations to trial. According to the company, 130 billion executions have now been run on the Q Network, and 100 commercial partners, including Delta Airlines, Goldman Sachs, and ExxonMobil, have signed up for membership.
- Rigetti launched it’s cloud computing service in 2018, along with a $1 million prize for the first conclusive demonstration of quantum advantage on their platform. (Note: his is currently a quantum simulator, rather than access to a full device.)
- D-Wave has launched cloud services, as well as selling quantum annealing hardware.
- AWS is offering access to others’ quantum computing services through its quantum solutions platform.
While other business models like hardware or equipment sales and consulting services are possible, we expect QCaaS to be the main source of revenue based on scalability. That said, several quantum computing companies hope to secure profit-sharing contracts similar to the relationship between 23andMe and GlaxoSmithKline, through which 23andMe receives 50% of the profits from any GlaxoSmithKline drug for a target identified using data from 23andMe’s health app. The value of the quantum computer and quantum software tools in creating the final product will certainly be the topic of heated contract discussions.
Quantum Software
While outside the scope of Prime Movers Lab investment, software development for quantum computers is also a growing area, with well over a dozen startups focusing on providing software services (notably Cambridge Quantum Computing, 1QBit, Strangeworks, and Zapata).
Macro
Market Sizes & Stats
The quantum computing market is anticipated to grow from $472 million USD in 2021 to 1.765 billion USD by 2026 (source: Quantum Computing Market with COVID-19 impact by Offering (Systems and Services), Deployment (On Premises and Cloud Based), Application, Technology, End-use Industry and Region - Global Forecast to 2026), at a CAGR of 30.2% over the forecast period.
The early use of quantum computing in the banking and finance industry is projected to boost the market's worldwide expansion. Other important factors driving the growth of the quantum computing market include increased government funding in research and development activities connected to quantum computing technology. Following COVID-19, some firms are concentrating on the implementation of Quantum Computing As a Service (QCaaS). This, in turn, is likely to help the quantum computing business develop. However, difficulties with stability and error correction are anticipated to limit market development.
Quantum computing is gaining popularity in the banking and finance services business, which is concentrating on accelerating trade operations, transactions, and data processing by orders of magnitude. Simulation is one of the most important possible uses of quantum computing. Quantum computing aids in the discovery of a more effective and economical method of managing financial risks. When conventional computers are employed in financial institutions, the processing time and costs of high-quality solutions can rise exponentially, but quantum computers can perform fast operations at optimal costs, resulting in cost reductions and new income potential.
Other markets that will drive the adoption will be healthcare and pharmaceutical, transportation and logistic, chemistry and material science, cybersecurity, academia, and governments.
Investment Snapshot
The number of companies founded steadily increased since 2011 and peaked in 2018 as can be seen in Figure 10.
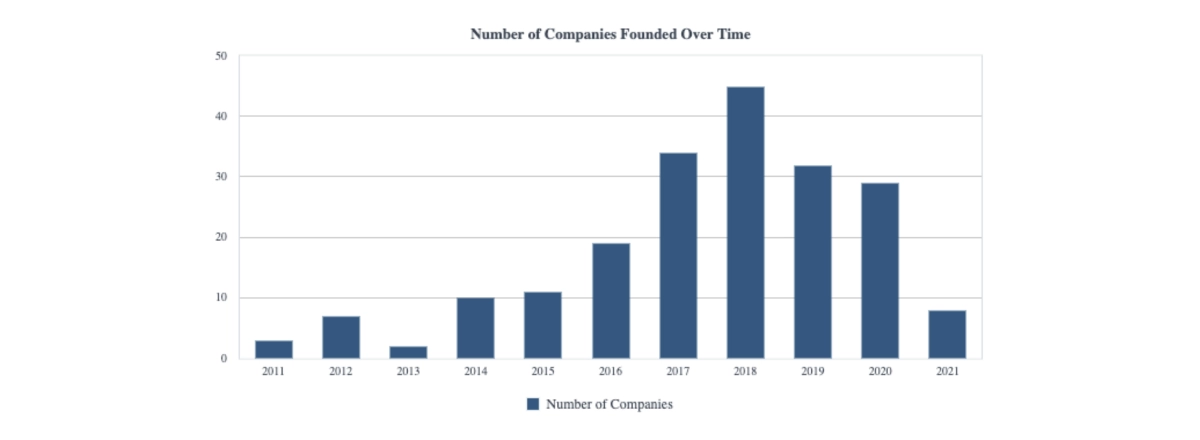
Figure 10. Number of companies founded over time. Source: Pitchbook
The number of quantum computing deals peaked in 2020 at 125. However, 2021, a record-breaking year for VC in general, saw the greatest deal value, with over $3B in quantum computing related deals as seen in Figures 11 and 12.
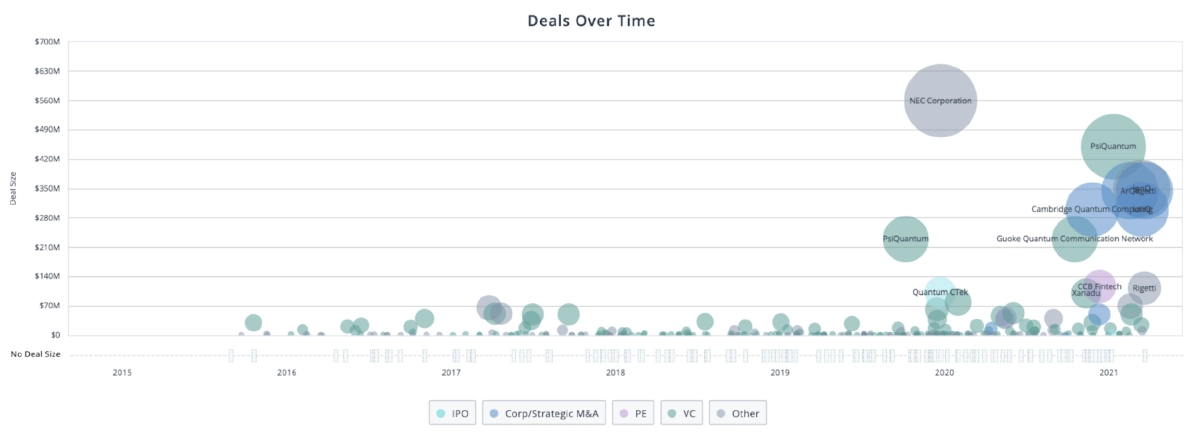
Figure 11. Number of deals over time. Source: Pitchbook
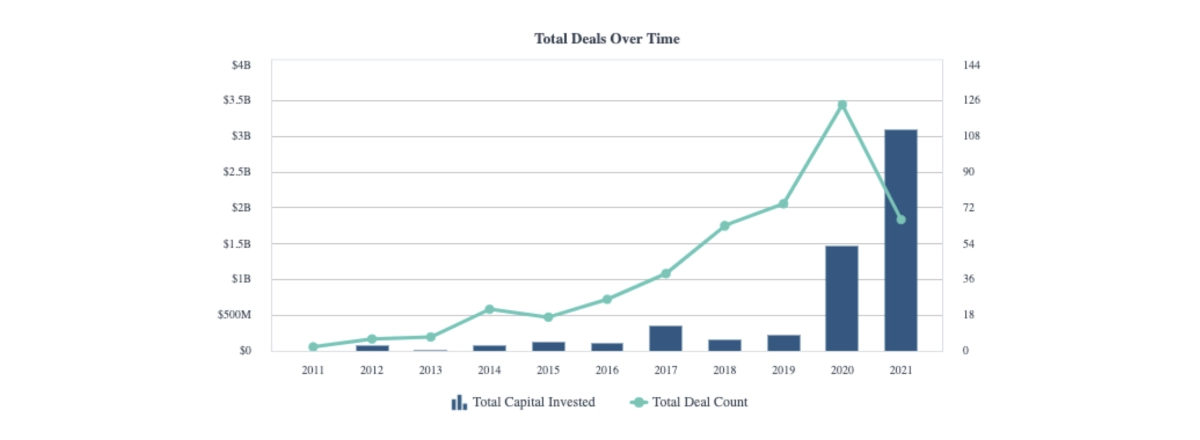
Figure 12. Investment value of deals over time. Source: Pitchbook
The year 2021 also saw some corporate and M&A activity in quantum computing for the first time.
IP Snapshot
The U.S. leads the world in the number of patent families related to quantum computing, according to pitchbook research. Roughly 60 percent of patents were filed in North America, 22 percent in Asia, 15 percent in Europe, and 2 percent in other continents (Figure 13).
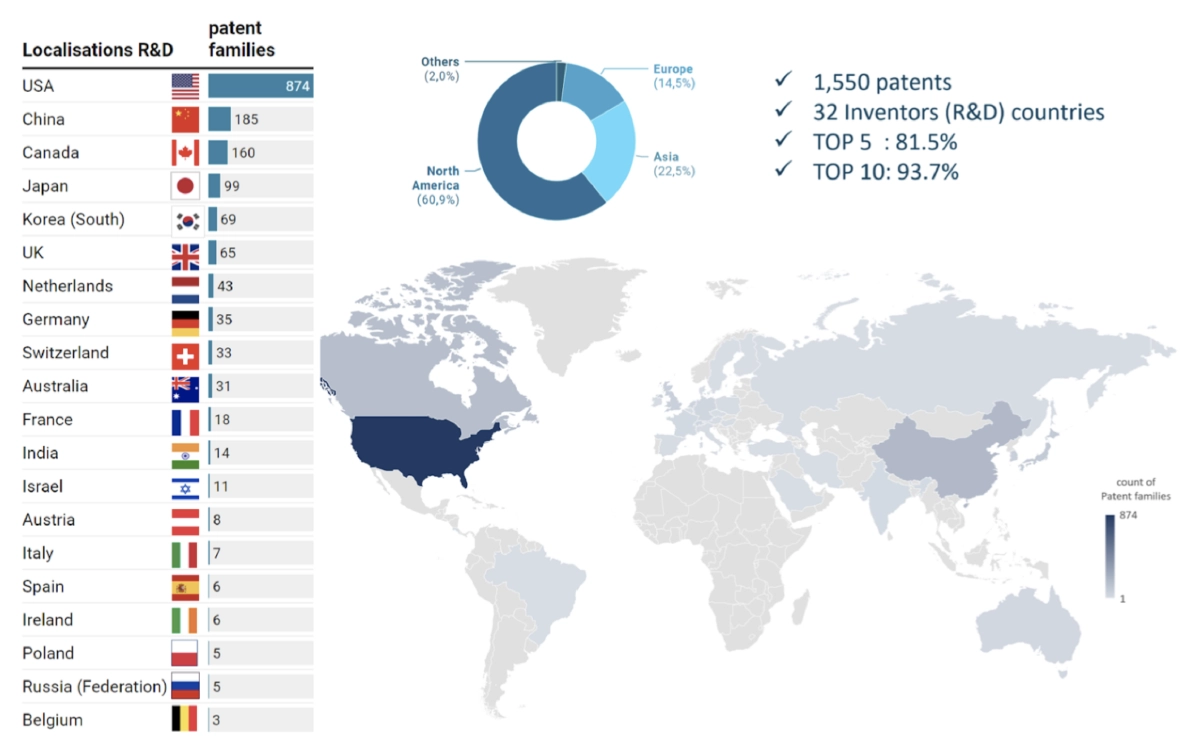
Figure 13. Location of patents by country of priority and location of the inventor.
Source: Quantum technologies patents, publications, and investments, Michel Kurek
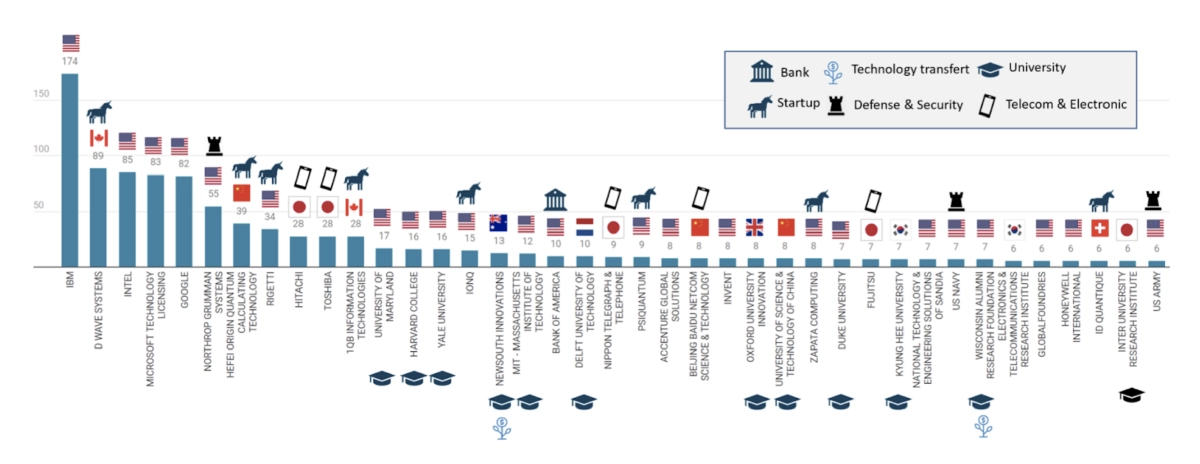
Figure 14. Organizations holding six or more patents since 2010.
Source: Quantum technologies patents, publications, and investments, Michel Kurek
We see several types of players on the list of IP holders (Figure 14). Of note:
- US IT behemoths (IBM, Intel, Microsoft, Google)
- Asian conglomerates, primarily Japanese, historically focused on telecommunications and electronic equipment (illustrated by a mobile phone on the graph: Hitachi, Toshiba).
- Startups specializing in quantum computing have built or intend to build a quantum computer (D-Wave, Rigetti, Origin Quantum, IonQ, PsiQuantum, Atom). Others are more concerned with software (e.g., 1QBit, Zapata) or communication security (Quantum ID).
- Defense and security sector organizations (Northrop Grumman on the corporate side, U.S. Navy, and U.S. Army on the military side) are also present, as are several civilian agencies and government research institutions.
Investing Thoughts
Landscape
A landscape look at quantum computing hardware companies
DWave
Amount Raised / Valuation: $227.63M / N/A
Technology: Quantum annealing algorithm and quantum computers
Applications: Quantum computers
Year Founded: 1999
Atom Computing
Amount Raised / Valuation: $21M / $51M
Technology: Trapped neutral atom
Applications: Quantum computers
Year Founded: 2018
ColdQuanta
Amount Raised / Valuation: $68.75M / $67M
Technology: Trapped neutral atom
Applications: High performance clocks, Quantum Radio frequency sensing, quantum positioning systems, quantum networking and systems
Year Founded: 2007
Honeywell Quantum Solution (HQS)
Amount Raised / Valuation: Publicly traded company. HQS will merge with Cambridge Quantum Computing
Technology: Trapped ion
Applications: Quantum computers
Year Founded: 1906
IonQ
Amount Raised / Valuation: $439.20M / $2B | SPAC on March 8, 2021 with a $350M PIPE
Technology: Trapped ion
Applications: Quantum computers
Year Founded: 2015
Universal Quantum
Amount Raised / Valuation: $4.44M / TBD
Technology: Trapped ion
Applications: Quantum computers
Year Founded: 2018
Rigetti
Amount Raised / Valuation: $186M / $129M
Technology: Superconducting qubits
Applications: Quantum computers
Year Founded: 2013
IBM
Technology: Superconducting qubits
Applications: Quantum computers
Google
Technology: Superconducting qubits
Applications: Quantum computers
IQM Finland
Amount Raised / Valuation: $75.44M / 26.54M
Technology: Superconducting qubits
Applications: Quantum computers
Year Founded: 2018
Oxford Quantum Circuits
Amount Raised / Valuation: $2.61M / $4.23M
Technology: Superconducting qubits
Applications: Quantum computers
Year Founded: 2017
PsiQuantum
Amount Raised / Valuation: $744.70 / $3.5B
Technology: Silicon photonic
Applications: Quantum computers
Year Founded: 2016
Photonic
Amount Raised / Valuation: $141M / $400M
Technology: Silicon photonic
Applications: Quantum computers
Year Founded: 2016
Xanadu
Amount Raised / Valuation: $141M / $400M
Technology: Silicon photonic
Applications: Quantum computers
Year Founded: 2016
EeroQ
Amount Raised / Valuation: Screened company - we know that they closed a round
Technology: E-He
Applications: Quantum computers
Year Founded: 2016
Microsoft
Technology: Topological
Quantum Brilliance
Amount Raised / Valuation: $10.92M / N/A
Technology: Diamond
Applications: Quantum computers
Year Founded: 2019
Deals
Recent deals in the quantum computing space
Rigetti (NYS: RGTI)
Deal Date: Oct 6, 2021
Deal Type: Reverse Merger
Deal Size: $345M
Post Valuation: $1,500M
Deal Status: Announced/in progress
Financing Status: Pending transaction (VC)
Business Status: Generating revenue
HQ Location: Berkeley, CA
IonQ (NYS: IONQ)
Deal Date: Sep 30, 2021
Deal Type: Reverse Merger
Deal Size: $300M
Post Valuation: $2,000M
Deal Synopsis: Acquired dMY Technology Group (NYSE: DMYI.U) through a reverse merger, resulting in the combined entity trading on the New York Stock Exchange under the ticker symbol IONQ
Deal Status: Completed
Financing Status: Corporation
Business Status: Generating revenue
HQ Location: College Park, MD
ArQit (NAS: ARQQ)
Deal Date: Sep 3, 2021
Deal Type: Reverse Merger
Deal Size: $345M
Post Valuation: $1,000M
Deal Synopsis: Acquired Centricus Acquisition (NAS: CENH) through a reverse merger for $345 million, resulting in the combined entity trading on the Nasdaq Stock Exchange under the ticker symbol ARQQ, putting the company's pre-money valuation at $655 million
Deal Status: Completed
Financing Status: Formerly VC-backed
Business Status: Generating revenue
HQ Location: London, United Kingdom
Zurich Instruments
Deal Date: Jul 1, 2021
Deal Type: Merger/Acquisition
Deal Synopsis: Acquired by Rohde & Schwarz for an undisclosed amount
Deal Status: Completed
Financing Status: Formerly accelerator/incubator backed
Business Status: Generating revenue
HQ Location: Zurich, Switzerland
Quantum Generative Materials
Deal Date: Jun 24, 2021
Deal Type: Merger/Acquisition
Deal Size: $50M
Post Valuation: $100M
Deal Synopsis: Acquired by Comstock Mining (ASE: LODE) for $50 million
Deal Status: Completed
Financing Status: Corporate backed or acquired
Business Status: Generating revenue
HQ Location: Cheyenne, WY
Cambridge Quantum Computing
Deal Date: Jun 8, 2021
Deal Type: Merger/Acquisition
Deal Size: $300M
Post Valuation: $545.45M
Deal Synopsis: Reached a definitive agreement to be acquired by Honeywell (NAS: HON)
Deal Status: Announced/in progress
Financing Status: Pending Transaction (M&A)
Business Status: Generating revenue
HQ Location: Cambridge, United Kingdom
Quantum Benchmark
Deal Date: May 25, 2021
Deal Type: Merger/Acquisition
Deal Synopsis: Acquired by Keysight Technologies (NYS: KEYS) for an undisclosed amount
Deal Status: Completed
Financing Status: Formerly VC-backed
Business Status: Generating revenue
HQ Location: Kitchener, Canada
Muquans
Deal Date: May 20, 2021
Deal Type: Merger/Acquisition
Deal Size: Acquired by iXBlue for an undisclosed amount
Deal Status: Completed
Financing Status: Corporate backed or acquired
Business Status: Profitable
HQ Location: Talence, France
High Precision Devices
Deal Date: Oct 19, 2020
Deal Type: Merger/Acquisition
Deal Size: $17M
Post Valuation: $17M
Deal Synopsis: Aacquired by Formfactor (NAS: FORM) - the acquisition expands Formfactor's cryogenic test capabilities
Deal Status: Completed
Financing Status: Corporate backed or acquired
Business Status: Generating revenue
HQ Location: Boulder, CO
Labber
Deal Date: Mar 2, 2020
Deal Type: Merger/Acquisition
Deal Synopsis: Acquired by Keysight Technologies (NYS: KEYS) for an undisclosed amount - the acquisition will enable the acquirer to explore new opportunities and drive next-generation innovation in quantum technology
Deal Status: Completed
Financing Status: Formerly accelerator/incubator backed
Business Status: Generating revenue
HQ Location: Vaxjo, Sweden
QxBranch
Deal Date: Jul 11, 2019
Deal Type: Merger/Acquisition
Deal Synopsis: Acquired by Rigetti for an undisclosed amount
Deal Status: Completed
Financing Status: Formerly VC-backed
Business Status: Generating revenue
HQ Location: Washington, DC
Quantum Computing (NAS: QUBT)
Deal Date: Jul 3, 2018
Deal Type: Reverse merger
Deal Synopsis: The company acquired Innovative Beverage Group Holdings (OTC:IBGHD) through a reverse merger resulting in the combined entity trading on the OTC stock exchange
Deal Status: Completed
Financing Status: Corporation
Business Status: Generating revenue
HQ Location: Leesburg, VA
Active Growth Capital
Deal Date: Nov 23, 2016
Deal Type: Merger/Acquisition
Deal Synopsis: Acquired by Quantum Numbers through a reverse merger, resulting in the combined entity trading on TSX Venture Exchange
Deal Status: Completed
Financing Status: Corporate backed or acquired
Business Status: Generating revenue
HQ Location: Brossard, Canada
Quantum Numbers (TSX: QNC)
Deal Date: Nov 23, 2016
Deal Type: Reverse merger
Deal Synopsis: Acquired Active Growth Capital through a reverse merger, resulting in the combined entity trading on TSX Venture Exchange
Deal Status: Completed
Financing Status: Corporation
Business Status: Generating revenue
HQ Location: Brossard, Canada
BBN Technologies
Deal Date: Oct 26, 2009
Deal Type: Merger/Acquisition
Deal Size: $350M
Post Valuation: $350M
Deal Synopsis: Acquired by Raytheon (NYSE: RTN)
Deal Status: Completed
Financing Status: Formerly PE-backed
Business Status: Profitable
HQ Location: Cambridge, MA
BBN Technologies
Deal Date: Aug 1, 1997
Deal Type: Merger/Acquisition
Deal Synopsis: Acquired by Genuity for an undisclosed amount
Deal Status: Completed
Financing Status: Formerly VC-backed
Business Status: Profitable
HQ Location: Cambridge, MA
For years, the quantum market seemed boring and sleepy even considering the eight M&A deals from 2016-2020. These deals were related to companies developing technologies for the quantum era encryption (Quantum Numbers), software for quantum equipment (Labber acquired by Keysight Technologies) but also software (Quantum Computing, QxBranch acquired by Rigetti, Quantum Benchmark acquired by Keysight Technologies) and HW components (High Precision Devices, Muquans) to be used with quantum. In 2021, there was a change in pace and also a change in the type of companies that were subject to SPAC or M&A. Eight deals happened in 2021. That is a sign of maturity in the industry that can now “show” early products. These products are still very early, with limited functionalities, i.e. they can solve very few computational problems.
Our perspective is that quantum companies are starting to be confident that they will be able to scale their architecture by increasing the number of qubits. For example, Rigetti announced a SPAC, claiming that their booking order went up to $15 million from the previous forecast of $5 million. Different components of quantum computing and software reached a good enough level to enable some of these quantum technologies platforms. Software is very strategic as confirmed by the acquisition of QxBranch by Rigetti. Also, the merger of Cambridge Quantum Computing and Honeywell Quantum Solution shows the same pattern.
Conclusion
Developing quantum computers involves beautiful math, breakthrough physics, creative engineering design, and enough uncertainty to capture the imagination. The key challenge for early-stage and growth investors alike is that the killer applications for quantum computers are still largely unknown.
The quantum computing field is growing up rapidly, but is still many years away from achieving a universal quantum computer. Quantum computers will need at least 1000 logical qubits to solve interesting problems, or about one million actual, physical qubits given expected error rates. By contrast, today’s most advanced quantum computer prototypes have at most 100 physical qubits.
Meanwhile, powerhouse companies like Microsoft, IBM and Amazon have begun to build out quantum service platforms in the cloud. This puts these megacompanies in a strong position to capture a large part of the value created, regardless of which quantum computing approach turns out to be the most powerful, useful and/or widely applicable. Quantum computing will be primarily offered as a cloud service, at least at first.
Quantum computers will one day have an advantage in certain classes of chemistry, materials science, and optimization routines. While there has yet to be a “killer app” for quantum, we suspect that it will come in the form of an optimization routine that generates value each time it is run. (It’s easy to imagine a future where some logistics problems are run on AWS “quantum cores.” It is less easy for us to imagine a future where a quantum computing company receives a massive payout for helping identify a drug candidate, although this business model is often pitched (a la the agreement between 23andMe and GSK to share profits from discoveries made mining 23andMe’s data).
Is this a challenging area for private investment? Yes, especially given that most experts in the industry predict that useful quantum computers are still 15+ years away. However, we feel it is worth the risk given the transformative nature of the technology. In addition to the classes of problems that we currently know quantum computers will excel at, more and more research is being done on areas where quantum computers (or even NISQ devices) can solve currently intractable problems. Research into combining quantum computing with machine learning and neural networks is especially interesting.
The rate of future progress in quantum computing could also accelerate rapidly. Major strides are being made in adjacent technology areas: high-temperature superconductors, optics, lasers, control systems, laser controls, and computation power. Scientific breakthroughs in quantum computing must be accompanied by breakthroughs in mathematics and computer science. For this reason, it is worth tracking progress in algorithm development, and in research on Noisy Intermediate Scale Quantum computers, or NISQ devices.
There is also downside protection in the tremendous amount of IP and know-how being generated by startups in this space. We are cautiously bullish on this space, especially for quantum computing technologies that have the ability to scale the number of qubits rapidly, have a pathway to high fidelity, and show strong customer focus early.
While it isn’t too late for new startups to make a splash, the wave of new quantum company formation is slowing down. University spinouts are common in the field of quantum computing startups, given that much of the IP generated in this space comes from a relatively small set of physics departments around the world. At this point, most of the more researched approaches to creating qubits are now being pursued by IT giants and/or startups.
Several quantum computing companies are now blazing trails in the public markets: Rigetti, IonQ, and Cambridge Quantum Computing to name a few. This has established that there is a path to exit for this sector, though it may prove to be a rocky one.
Perhaps the best argument that quantum computers will eventually become critical to new products is looking back at how people (specifically leaders in the field!) viewed classical computers in the early days. The inventors of the transistor and early computer startups could not have possibly imagined how navigation apps, internet search, online banking, video streaming, e-commerce, and social media would infiltrate and change modern life. Similarly, it’s hard to imagine what people might create with quantum computers. We want to conclude with a famous quote from the chairman of IBM Thomas Watson in 1943, “I think there is a world market for maybe five computers.”