Orbital Debris
Prime Movers Lab
April 2022
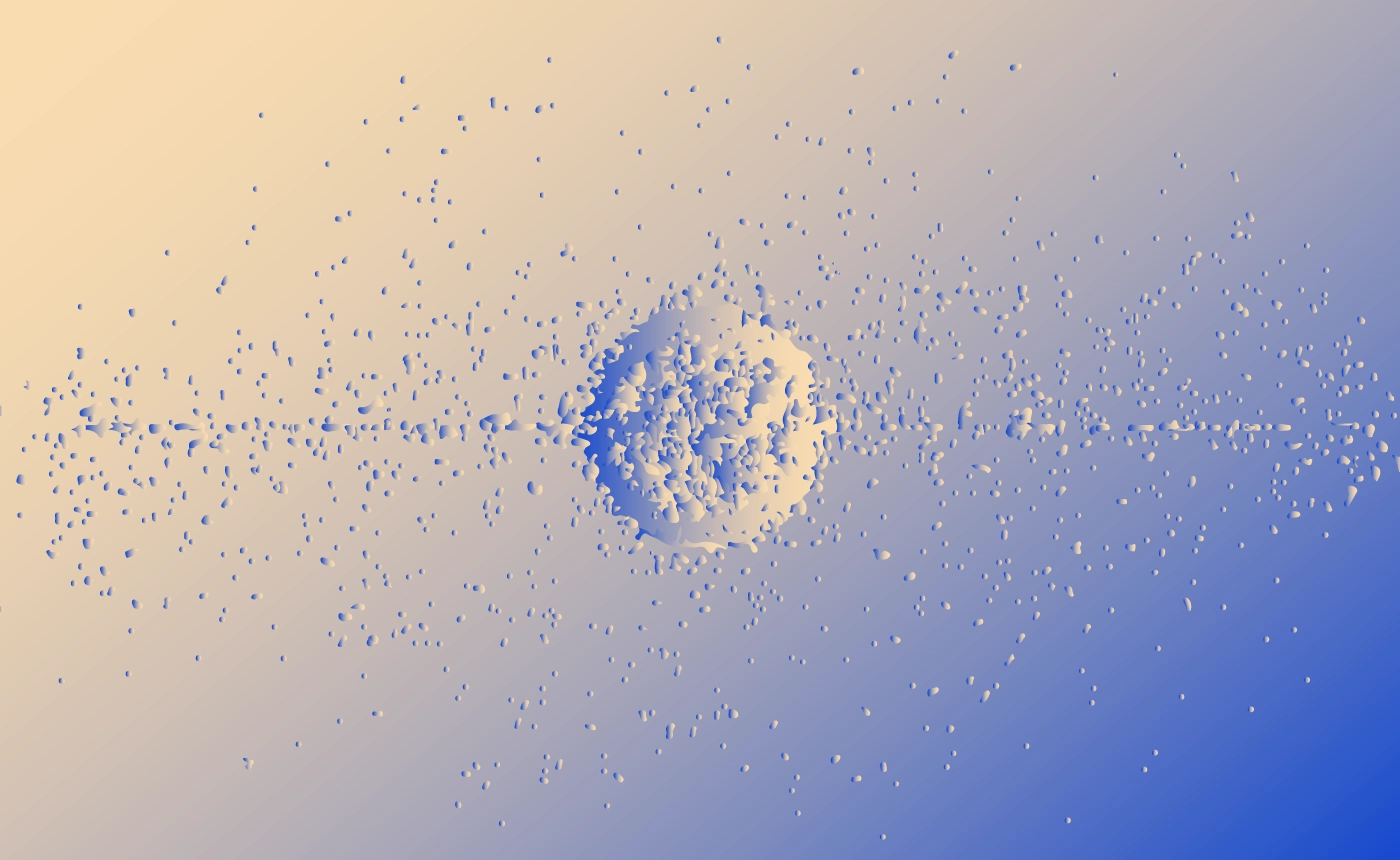
Introduction
Some high-value areas in orbit around Earth are getting crowded. The number of spacecraft launched per year has increased ten-fold compared to a decade ago. Statistics vary as references are quickly obsolete with each new launch (which alone reinforces that orbital content is increasing rapidly). Regardless, the trend does not appear to be slowing. The latest Euroconsult Analysis predicts a four-fold increase in satellite launches in the next decade – or approximately 17,000 new satellites.[1]
Much of the growth is fueled by the new low and medium earth orbit mega constellations, being brought to you by SpaceX (Starlink), Amazon (Kuiper), OneWeb, Telesat, E-space and China’s GW, to provide relatively inexpensive global internet services. Meanwhile, behemoth communication satellites in geostationary orbits that provide continuous wide-spectrum coverage to an entire continent are not going away.
No traffic lights exist on these orbital highways. These operational assets must track traffic in their “neighborhood” and decide whether to take action to ensure they don’t crash. Add uncontrolled, defunct satellites and spent stages of launch vehicles as obstacles on the road. Then literally pepper the expanse with shards from explosions caused by residual fuel, thermally cycled batteries, or in-space collisions. These millions of smaller pieces go whizzing in and out of orbits given the velocity changes imparted when they were birthed by explosions or collisions.
At Prime Movers Lab, we have a deep interest in creating a sustainable space environment for the healthy and prosperous growth of the space economy and infrastructure on which we all depend. To understand the dangers of growing congestion and orbital debris – and what we can do about it – we have spent time speaking with numerous companies developing orbital debris-related technologies and services as well as space environmental, insurance, policy, and law experts (see our webinar recap). What follows is a summary of what we have learned.
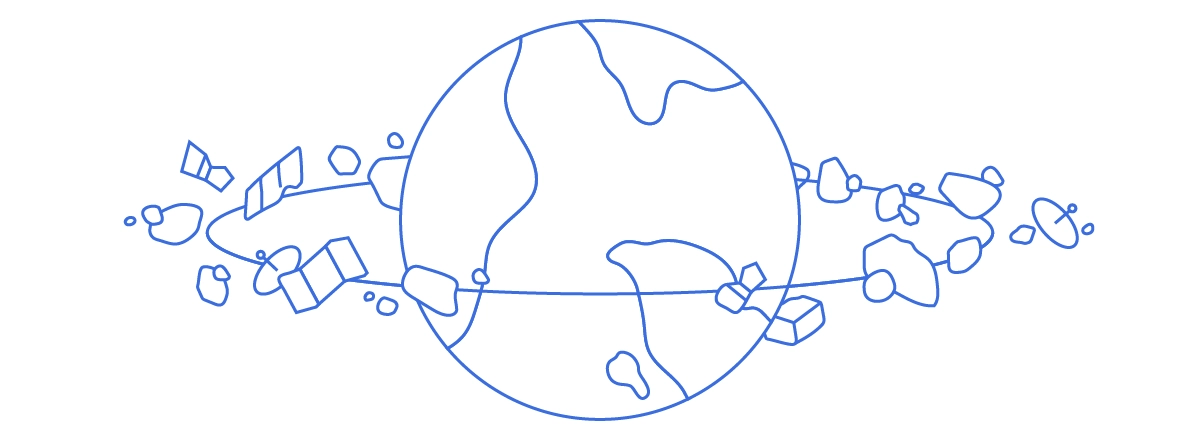
The Threat
To illustrate the magnitude of threats, consider these figures updated monthly by ESA.[2] There are about 5,200 currently-functioning satellites today. There are over 2,600 additional inactive satellites still in space. Then there are almost 30,000 debris objects larger than 10 cm (or about a softball) regularly tracked and cataloged by the U.S. Space Surveillance Network for collision avoidance purposes.
As of last 2021, one third of these objects were attributed to two events:[3] China’s anti-satellite test (ASAT) in 2007, and an accidental collision in 2009 between a retired Soviet Union-era satellite and an operating U.S. spacecraft. These events demonstrate another issue – not only the plethora of debris created in the first place, but its persistence over a decade later.
The threat of more events like these is increasingly frequent, including the near-miss of two obsolete spacecraft reported last year[4] – neither of which can maneuver to avoid a collision, and the recent ASAT conducted by Russia.[5]
But wait – there’s more! Statistical models estimate another 7,000 objects larger than 10 cm are not tracked.
That is a lot of numbers. Let me be clear: Over 44,000 objects of at least the size of a half brick, whizzing by at orbital speeds.
Just as dangerous, statistical models estimate there are nearly one million objects between 1 cm to 10 cm and over 130 million objects less than 1 cm. These projectiles move at speeds faster than bullets and can wreak havoc.
To get a sense of all the debris orbiting the Earth, see the ESA 2019 orbital debris animation[6] (set playback speed to 2x and note the color key to the right).
Consequences are real: NASA’s space shuttle has considerable evidence of the damage caused by even the tiniest orbital debris, requiring costly repairs and threatening astronaut lives. The International Space Station maneuvered or sheltered their crew at least three times in early 2022 in three months alone.[7-9] The increasing threat of orbital debris, especially given the rise in mega constellations, prompted NASA to officially and publicly voice concerns to the Federal Communications Commission (FCC).[10]
A little higher up, we also learned the break-up of a Chinese satellite was caused by a small piece of debris from a 1996 Russian launch vehicle.[11] And satellites in geostationary orbit are just as vulnerable, which can disrupt service and revenue, and increases capex (see the tracking video that captures the anomaly taking out a telecommunications satellite after only a fraction of its service life[12]).
The threat is not contained to in-space assets. As early as 1997, a woman was hit by what is assumed to be a piece of a Delta II rocket falling to Earth.[13] In 2021, a Falcon 9 second stage pressure vessel (about one and a half meters long) landed in Washington state (it was launched from Florida).[14]
The threat will, unfortunately, continue to grow with the deployment of multiple constellations, the decreasing costs of satellites, the decreasing costs of launches, and the inflection of in-space commercial space stations. With such an exponential increase in population, a collision could create a cascading effect – known as the Kessler syndrome – as more debris is created and collides with neighboring spacecraft or debris. This chain reaction could wipe out parts of the space infrastructure for years to come. Disruptions would be felt globally. Myriad industries, governments, and militaries rely on the space infrastructure for cellular communications, Global Positioning System (GPS) navigation, weather and climate monitoring, and data transmission. In fact, so much so, the U.S. is considering establishing space as critical infrastructure under the Space Infrastructure Act.[15]
Solutions
Ok. So lots of objects are whizzing around in space. What do you do about it?
Tracking and Warnings
The first step is to track the objects and warn of “conjunctions” (when two objects pass near each other).
Conjunction warnings are communicated via industry-standard Conjunction Data Messages (CDM). CDMs require operators to make risk assessments on whether to implement collision avoidance maneuvers. In other words, should they use resources to move their satellite? This is a complicated decision, trading the chance of a collision with reduced mission life and revenues.
Evaluating the chance of collision is complicated due to position uncertainties. (i.e. Do you really know where the two objects are in space?) Knowing exactly where an object is in space is tremendously difficult, especially given orbital perturbations such as Earth’s dynamic atmosphere that can grab at orbiting objects, the gravitational pull of the sun and moon, and irregularities in Earth’s gravity based on the Earth not being a perfect sphere. Debris can be even more difficult to pinpoint due to a lack of accurate knowledge regarding its mass and the area upon which these perturbations act. Position uncertainties can range up to 100 m for a spacecraft and over 1 km for a piece of debris. These uncertainties accumulate and then grow as an object is propagated in time. They can be represented as error ellipsoids or “covariances.” Reducing these covariances can be achieved by using multiple data sources. When the covariance of one object intersects the covariance of another - that is when you should worry.
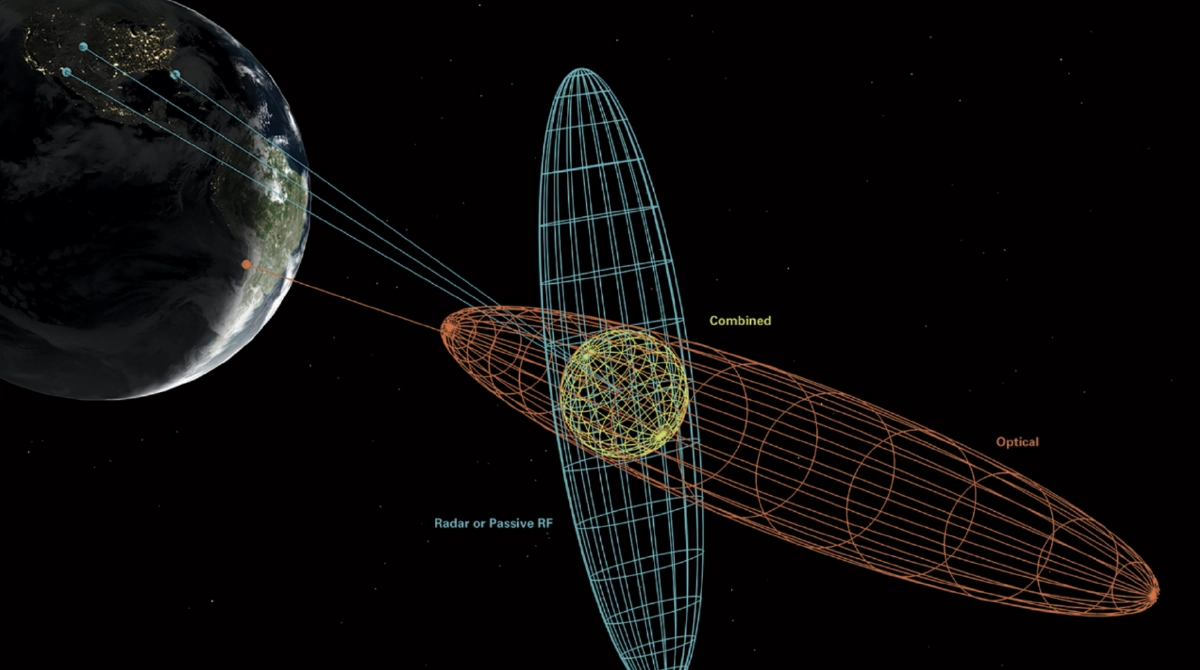
Combining multiple data sources can reduce uncertainty ellipsoids, or covariances, around an object. Image credit: AGI Orbit Determination Tool Kit (ODTK)[16]
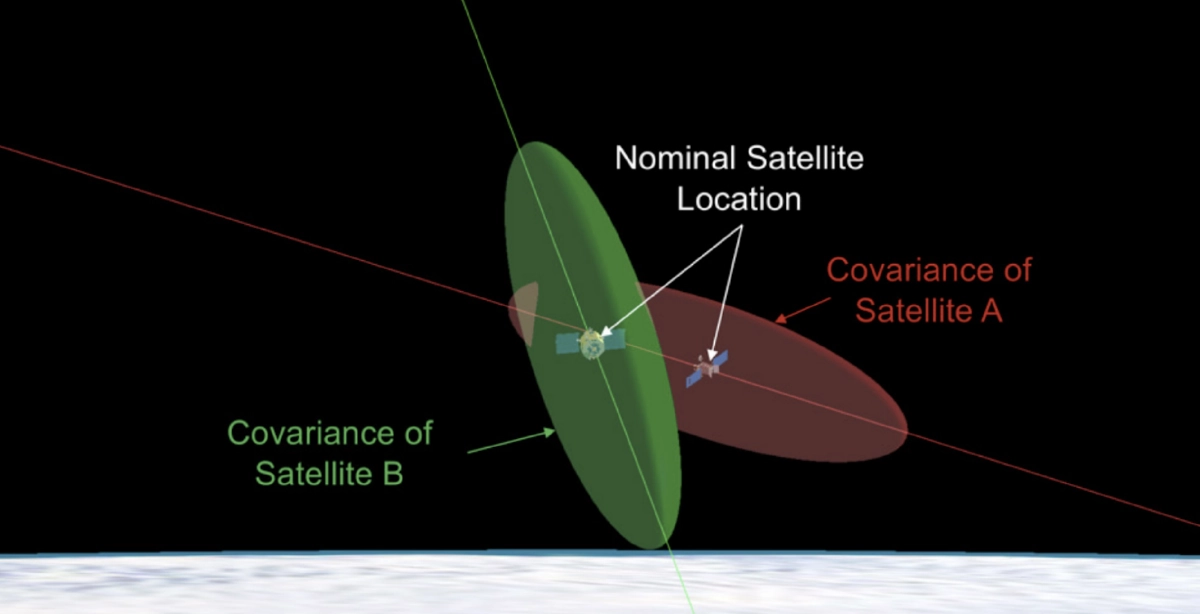
Covariances intersect along orbital paths, triggering a conjunction warning. Image credit: The Aerospace Corporation[17]
Conjunction warnings obviously consume the time and attention of spacecraft operators, who need to consider the risks of action versus inaction. Inaction (or not maneuvering) could obviously result in a collision, losing an asset and creating more debris. Action consumes resources which may reduce mission life, revenues and customer satisfaction. For example, consuming propellant for an unplanned maneuver to avoid space junk could shorten a mission. As you deplete your propellant, the amount of “station keeping” that you can do also reduces. Meaning a five- year mission life might be reduced to four years because you can’t maintain the position in orbit required to deliver your services. Reduced time on station means reduced time to generate revenue for that asset. Add to that the possibility that maneuvering your asset may temporarily cut services which could impact revenues further (and likely make some customers unhappy).
How often do CDMs occur? The number of conjunctions between two operational spacecraft at < 1 km apart (a probability of collision greater than 1E-6 or one in a million) currently occurs around 3,200 times per week[18] and is growing. When you consider conjunctions involving trackable debris, one study suggests that number grows to over 27,000 a week.[19] The study makes a case that conjunctions internal to a constellation (such as a conjunction between two Starlink satellites) are negligible risk due to the “robust operational stationkeeping and collision avoidance capability exhibited to date.” Think of this as a well-coordinated marching band performance at a football game’s halftime.
Starlink inter-constellation conjunctions accounted for 45 percent of the total identified conjunctions. No other mega constellation has this much internal traffic management – yet. Regardless, if you discount the conjunction warnings within Starlink, the number of conjunctions remaining to assess is still staggering at over 14,000 a week. Within those, debris – the stuff you can’t control – completely dominates. Over two-thirds of all conjunction events in LEO are potential debris-on-debris collisions. Only LeoLabs monitors and characterizes these events on a daily basis to provide perspective on likely future accidental debris-generating events.
Still conjunction warnings to which SpaceX responds appear to also be from debris, responses which consume valuable resources. SpaceX reported in FCC filings that last year, its satellites, “which are equipped with automated collision-avoidance systems, conducted more than 5,500 moves, or about 15 a day. Most were related to debris or satellites that couldn’t be moved…”[20]
Note, the 2021 study discussed here used LeoLab’s impressive catalog of 16,852 objects where the smallest is on the order of “operational cubesats as small as 0.25U (10cm x 10cm x 2.5cm).”[21] (As of this writing, their LEO catalog is up to 19,511 objects.) It does not speak to the other 62 percent of the total assumed 44,000 plus objects greater than 10 cm beyond LEO.
So let’s dive a little deeper into tracking!
Ground-based Tracking
The United States has been tracking space objects since Sputnik in 1957, resulting in the Department of Defense's global Space Surveillance Network now operated by the U.S. Space Force. They employ phased-array radars, conventional radars, electro-optical sensors, and even an in-space-based Midcourse Space Experiment (MSX) satellite.[22] They share information with governmental, academic, and commercial partners from 25 nations. The Space Surveillance Network catalogs and identifies the objects tracked, and then attempts to notify operators of conjunctions. Last year, the Space Surveillance Network issued 20 million conjunction data messages.[23]
So, help from commercial entities like LeoLabs is welcome. LeoLabs also uses phased-array antennas, with improvements to enable deployment cost and schedule at a fraction of what the military can do and allow for down to 2 cm diameter object detection.[24] They currently have sites in New Zealand, Alaska, Texas, and Costa Rica and plans for two more, in the Azores and Australia.[25]
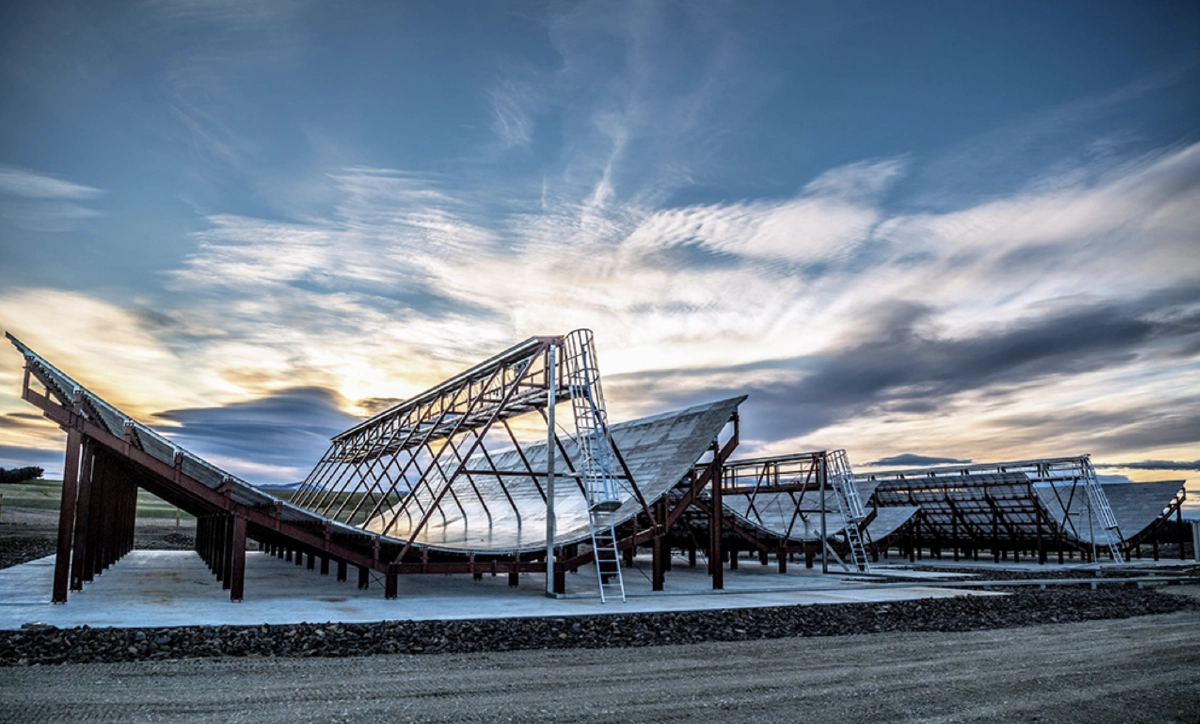
The LeoLabs Phased Array radar in New Zealand. Image credit: LeoLabs[26]
Space-based Tracking
Commercial options to track debris are coming online that can track from space. In-space observations have the potential for even greater resolution and lower uncertainties due to views unobstructed by the atmosphere (such as clouds, atmospheric attenuation, and scatter). They also may offer higher revisit rates (how many times a client is tracked in a given period). However, space-based sensor selection depends upon its resident orbit and their clients’ orbits, the power and real estate available on its host space-borne platform, and the clients’ characteristics (e.g., size, surface materials).
To appreciate the complexities, consider typical sensor choices for a space-based system. Active sensors emit radiation towards an object and measure reflection and backscatter. These include radars and LiDaRs. Passive sensors simply detect radiation, either emitted by the object (e.g. infrared radiation) or reflected by the sun (e.g. visible radiation). These include IR sensors in missile detection systems and optical telescopes. Thus, active sensors will require significantly more power than passive sensors.
Power requirements scale with the distance between the sensor and the object, determined by their positions within their relative orbits and changes over time. Further, some sensors may not work continuously, such as when an object moves into the shadow of the Earth (no longer illuminated by the sun).
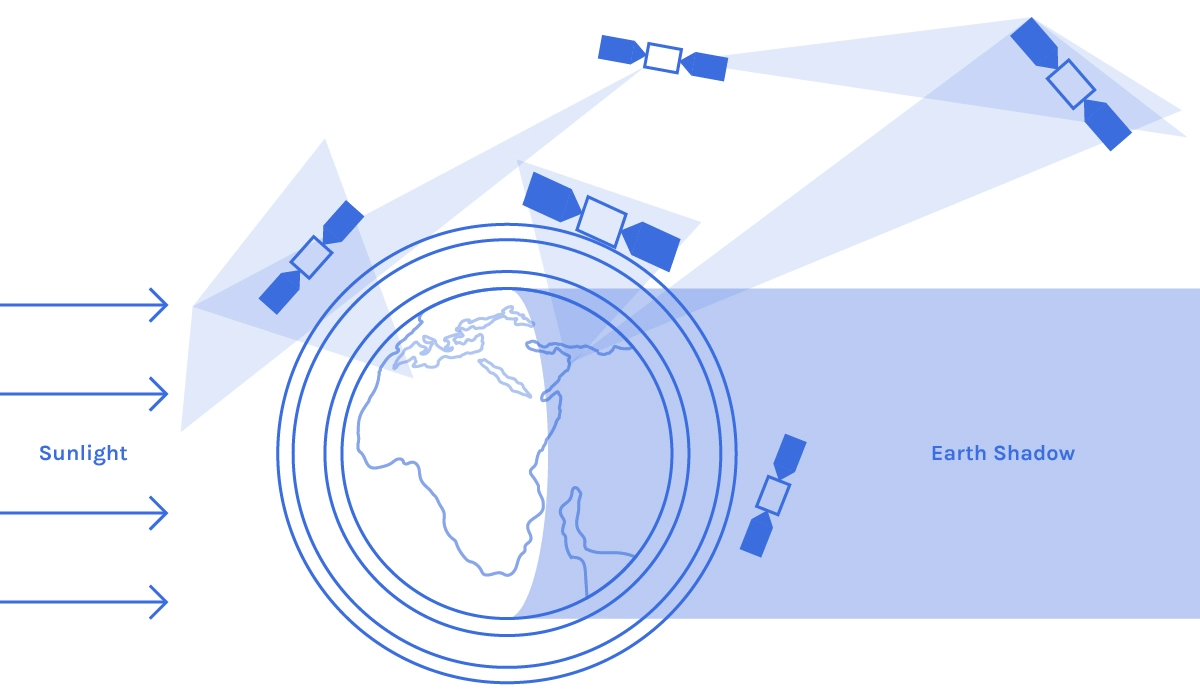
Optical space surveillance
As space-based tracking services take capital and time to build a constellation to provide robust tracking and catalog services, other services and products are being coupled to shore up the early business cases. These might include using the sensors to assist with inspection of nearby assets, performing rendezvous and docking to move or service an asset, or performing Earth observations.
SCOUT offers an example of high-resolution optical sensors for in-space observation data and services. Their SCOUT-Vision payload system uses computer vision coupled with guidance software to also enable autonomous operations. They promote that their customers (e.g. those providing on-orbit services to remove debris or extend mission lifetimes) will get 1000x better resolution for rendezvous operations. Their first SCOUT-Vision launched in June 2021. They also plan a fleet of in-space observation spacecraft called “OVER-Sats” that may collect data to aid in space domain awareness and space traffic management. SCOUT plans to launch its first OVER-Sat in 2023, and have services available late in 2024.
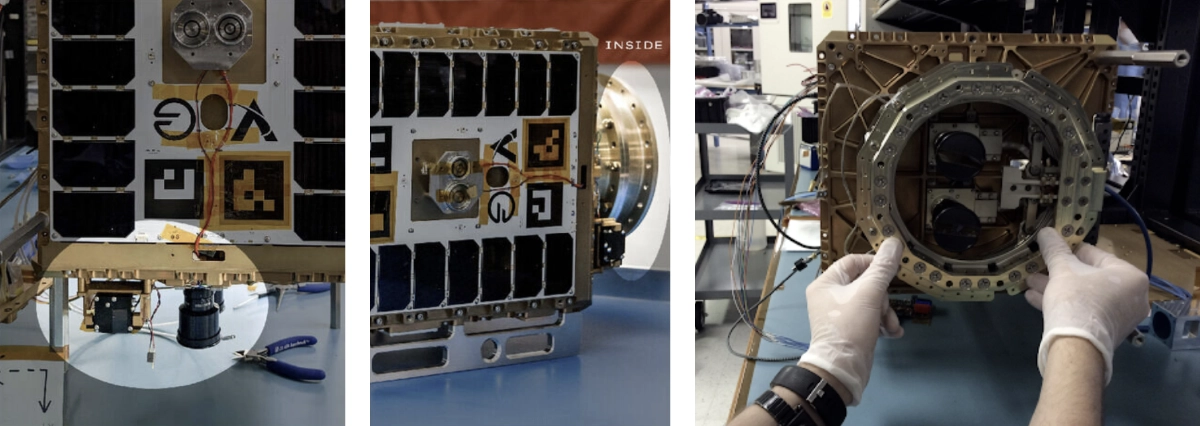
SCOUT-vision payload (noted by high-lighted areas) launched by SpaceX in June 2021 onboard Orbit Fab’s Tenzing-001 tanker. Image credit: SCOUT[27]
NorthStar Earth & Space, a Canadian company, also uses optical sensors to track objects from orbit. They plan a 52 satellite constellation in low earth orbit, 12 of which will use the optical sensors to enable a subscription model for traffic data and collision warning services by 2025. The remaining 40 will provide Earth observations using hyperspectral and infrared data. NorthStar also plans to offer multi-source data fusion, integrating various data sources to provide Earth environment monitoring products.
Like SCOUT, NorthStar will partner with satellites to host their sensors. NorthStar originally contracted LeoStella, Thales Alenia Space’s smallsat-manufacturing joint venture with BlackSky (NYSE: BKSY), to build and deploy their first three satellites by 2022. However, obtaining those licenses has been tricky since regulators treat the constellation as a whole (i.e., NorthStar can’t get separate licenses for the first three). If spectrum is allocated to (and thus consumed by) a constellation of 52 satellites, then regulators want to see a plan that includes milestones for the entire constellation deployed within nine years. That requires quite a bit of capital and early commitments!
In recent developments, NorthStar has partnered with Spire (NYSE: SPIR) to develop three satellites for launch in 2023 that will carry NorthStar sensors. Space situational awareness (SSA) data will be collected for NorthStar, but be operated as part of Spire’s “space-as-a-service” constellation.[29] A partnership was also recently announced between NorthStar and SES (EPA: SESG) to launch, develop and evolve NorthStar’s SSA products for use by SES’s satellite operations for fleet management.[30]
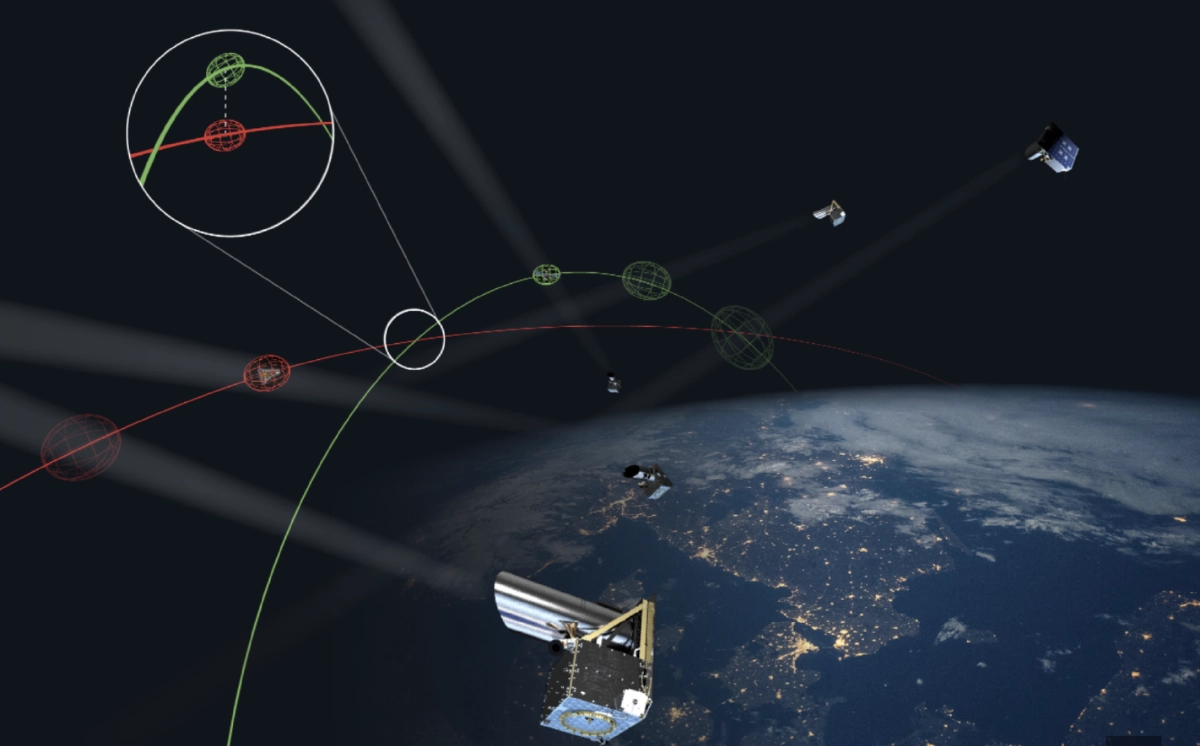
Northstar's constellation of satellites will use optical sensors for tracking objects across multiple orbits.
Image Credit: Northstar[31]
Multiple satellite sensors may be grouped to provide more robust coverage and specific characteristics of objects (in addition to their orbits). Recently formed, Privateer/[32] offers a constellation of multi-sensor satellites for debris location and characterization. They, too, are proposing to put their sensors on other spacecraft and leverage existing constellations. However, the real technology here may be a branch of Chief Science Officer and Space Environmentalist Dr. Moriba Jah's ATRIAGraph, called “Wayfinder”. Wayfinder is an open-access and near real-time visualization of objects in Earth orbit. They are offering object state information (position, velocity) for free, 24 hrs in advance; any predictors farther into the future than that would be for a price. They then provide an application on top of which people can build different services, for example, companies planning satellite servicing or debris removal activities.
Vyoma is building a satellite constellation that uses onboard optical cameras and global navigation satellite system (GNSS) receivers. The receivers allow Vyoma to precisely determine the orbit of their own satellites which then, with the optical data, are used to inform the location of the objects being tracked. They also incorporate data from ground-based sensors, and the latest self-calibrated and -maintained atmospheric models.
Vyoma sources both the spacecraft and optical sensors but intends to own/operate the constellation. They perform edge computing, using machine learning with orbit determination algorithms to reduce debris position uncertainties to < 100 m, real-time. The reduced data is then sent to the ground for physics-based modeling of atmospheric drag. Ultimately they claim tighter 3-day projections of positional uncertainty, reducing the number of times a satellite operator would have to maneuver. Like other providers, they intend to offer additional services to shore up the business case. For Vyoma, the differentiator may be providing third-party software that uses Vyoma observation data to automate satellite responses to conjunction warnings.
Debris Remediation
The next step to addressing the threat is through “remediation.” There are basically three methods: removal, rehabilitation, and recycling.
Removal
There are two classifications of debris removal: passive debris removal and active debris removal (ADR).
Passive debris removal takes no action and is thus “passive,” and it usually addresses the “small” stuff. These untrackable and hard-to-pinpoint objects are so small that a mission to actively collect a single piece wouldn’t be worth it. Technology to simultaneously address debris fields created, for example during an ASAT, would be of interest.
We have only spoken with one company in this space – Launchspace Technologies Corporation. They are proposing to deploy “Debris Impact Pads” to capture small debris in LEO-like catcher mitts. Currently, they are awaiting debris-generated impact data from a multilayer insulation blanket on the Space Systems Interface Flight Qualification Experiment (iSSIFQE), deployed last month outside of the International Space Station (ISS).[33] Launchspace will use the information to inform their own experiment to be placed on Airbus’ Bartolomeo exterior ISS platform in 2023.
Studying the small debris environment was done brilliantly by NASA’s Long Duration Exposure Facility (LDEF) mission. Launched in 1984, this huge 9+ meter long platform was covered with various materials and substrates and exposed to the orbital environment for over five years. LDEF resided in similar altitudes to ISS (approximately 400 km).
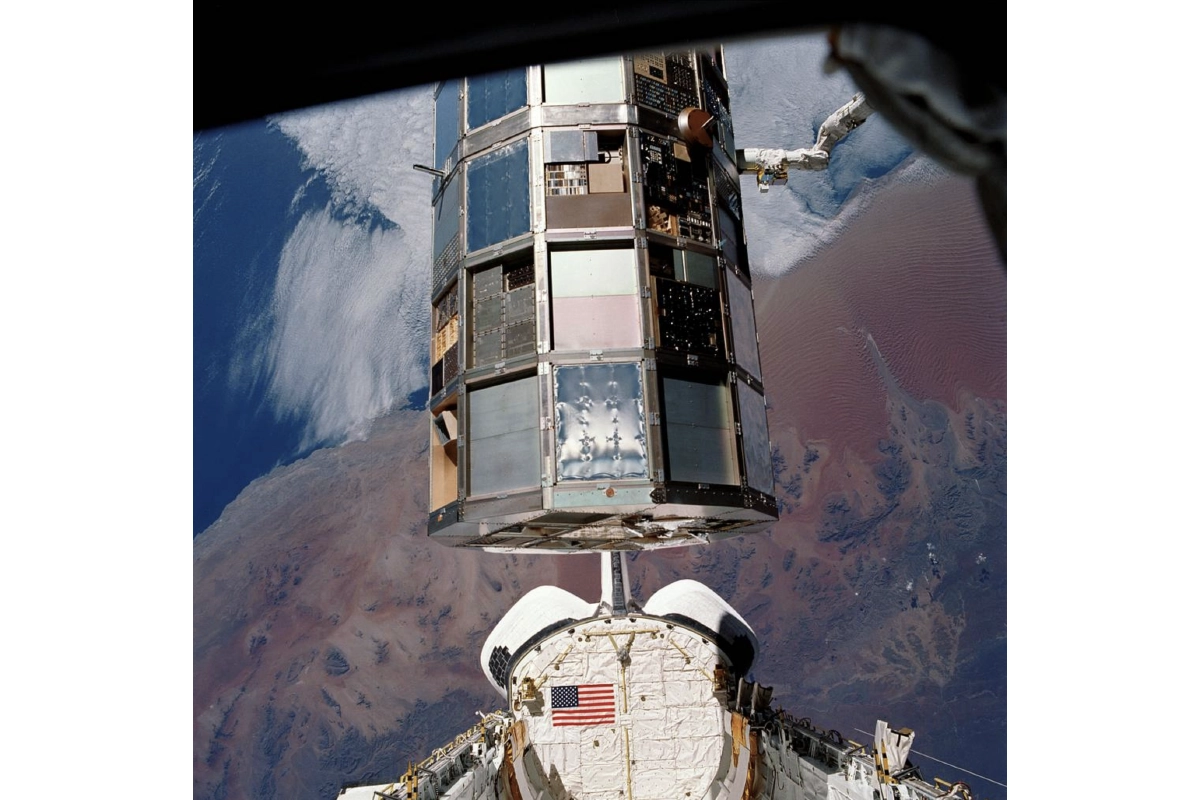
LDEF retrieval by STS-32 space shuttle mission in 1990. Image credit: NASA
The trick is to capture the debris without creating more debris. In LEO, small pieces can be traveling at speeds up to over 7,800 m/s (17,500 mph). At these speeds, “hypervelocity” impacts can cause materials to liquefy or even vaporize. Spall, fragments breaking off a larger body, can be ejected creating more debris. Even if the debris is caught and does not exit the other side, the impact can create spall on the backside as seen here.

Projectile impacting a thin aluminum plate at 7,000 m/s. The particle disintegrated and created fragmentation on the backside despite not penetrating the plate. Image credit: NASA, Public Domain
“Whipple shields” have been used successfully for quite a while to protect crew and spacecraft from these small hypervelocity impacts in space. Named for the inventor Fred Whipple, the shield consists of multiple layers. The first does little to stop the particle but rather acts to break up the debris and disperse the now smaller fragments. The many layers through which the particles pass are optimized with various structures and materials to minimize mass while entrapping the debris and spall. The innovation here is unlikely the pad but rather its size, deployment, operation, and business case to be effective.
ADR is taking action to locate, capture and remove a piece of debris. Usually ADR applies to large, tracked objects. With a catalog that contains thousands of candidates greater than 10 cm, the question is how to prioritize the debris.
One way is to consider which objects have the potential for generating the most debris. Two large objects colliding will generate more debris than two paint chips. Further, the probability of a collision between two large objects is greater than those of two paint chips. One can adjust the statistical risk of generating more debris due to a collision by multiplying the probability of a collision by the masses of the objects.
NASA studies have shown that removing five “high-risk” debris objects in LEO each year could at least maintain the current LEO-debris population.[34] That study was performed in 2011 - before the commercial explosion we are seeing now! So the number to take out annually to maintain the current debris field is likely larger – and greater still if we want to actually start cleaning it up.
LeoLabs publishes a “greatest hits” list (pun intended), based on the potential of a given object to create debris. The more debris-generating potential, the higher the chance it makes it on the list! Here I share their original top 50 list from 2019 that was confirmed in 2021 as still valid and part of their top 200.[19] They even identify from where the debris originates, which is critical because the 1967 Outer Space Treaty dictates that an object in space cannot be approached without permission from the responsible State.[35]
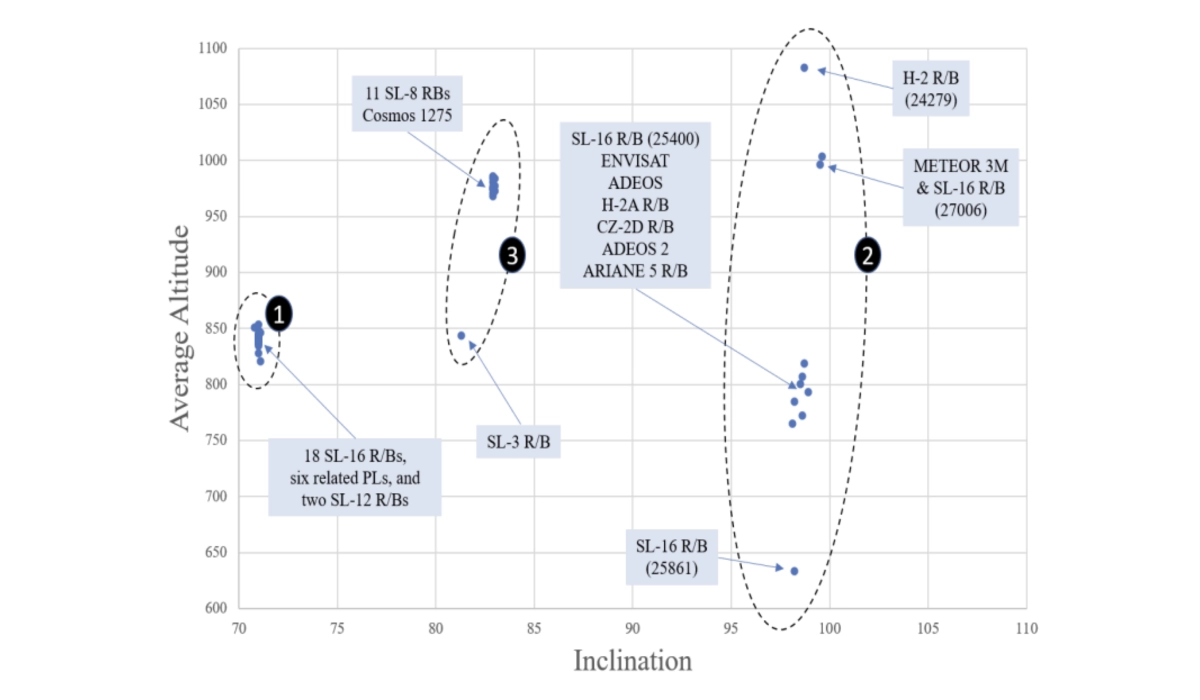
The top 50 statistically-most-concerning objects in LEO are largely within three groups. For example, the 850 km orbit around 71 degrees inclination hosts a cluster of derelik rocket bodies (R/Bs) and defunct satellite payloads (PLs).[36]
ADR can be accomplished by moving debris “into the atmosphere,” where the object may burn up upon reentry. This is accomplished by lowering the altitude of the object’s orbit: the lower the orbit altitude, the more the object dips into the upper layers of the atmosphere. Particles of the atmosphere hit the object’s surface, applying a force and decelerating the object. This lowers the object's velocity. The object can’t maintain its orbital altitude at the lower velocity and sinks further into the atmosphere.
The cost to move an object into the atmosphere increases with altitude. The higher the object, the less the atmosphere is able to “decay” its orbit. It may take only 25 years to decay an object at 700 km but 200 years to decay an object only 200 km higher. Best “practices” (note, not law) suggest an object should be removed within 25 years after its mission ends.[37] In cases where decay is longer than 25 years (and the operator wants to be a good samaritan), the natural decay may need an “assist” by applying a force on the object in the opposite direction of its travel, reducing the object’s velocity. Thus, the cost of a maneuver is measured in terms of the change in velocity required, delta-V (ΔV).
As an example, the force may be thrust applied via an engine by accelerating propellant mass (mp) along the direction of travel. How efficiently this happens is characterized by the specific impulse (Isp) of the engine. Delta-V can then be estimated based on a simplified rocket equation, where go is the gravitational constant of Earth and mo is the total mass of the object (including propellant) prior to the maneuver.
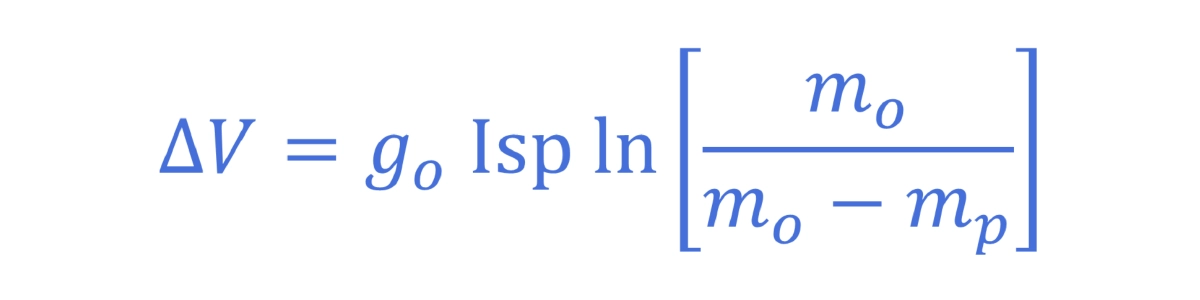
At some point, the cost to move the object higher into a “graveyard” orbit is less than moving it lower into the atmosphere. Graveyard orbits reside outside of operational orbits, typically about 300 km higher. For example, geostationary satellites (satellites whose orbit matches the rotation of the Earth such that they remain at the same spot above the Earth at all times) are at an altitude of 35,786 km. Assuming a Hohmann transfer maneuver (moving from one circular orbit to another circular orbit), it costs approximately 12 m/s of delta-V to raise the geosat into the graveyard. Alternatively, it would cost almost 1,500 m/s of delta-V to plunge the geosat into the atmosphere!
On-orbit services like tugs, or orbital transfer vehicles, can provide the delta-V needed to remove the debris. Grabbing or “grappling” these large objects can be tricky, especially if they are uncooperative and not actively controllable.
- The on-orbit service provider has to locate the object. They likely have their own search and tracking hardware and software, and they may augment with other tracking services to accelerate its operation and decrease uncertainties.
- The on-orbit service then has to rendezvous and dock with (or grapple) the object. This requires sophisticated guidance, navigation, and control sensors, compute power, and computer algorithms that can compute in real-time. The attitude control system has to be responsive enough to match any tumble rates of the object.
- Once captured, the on-orbit service has to dampen those rotational rates before setting the object on a safe, orbit-decaying course.
- All this without creating any new debris! Debris that could evolve if, for example, the service vehicle hit the client’s object during rendezvous or the service vehicle failed to grapple.
Passive mechanisms called grapple fixtures can be added to the design of a satellite (before it is launched) that will enable an on-orbit service to more easily grab the satellite and perform ADR.
Japanese startup Astroscale is an on-orbit service that uses magnetic grappling fixtures. Last year, Astroscale’s ELSA-d demonstrated a 175 kg “server” satellite releasing and capturing a client using such a fixture.[38] The demonstration mission, still active at the time of this writing, intends to end by disposing of the client into the Earth’s atmosphere, where it will incinerate. OneWeb, which has been adding magnetic grappling fixtures to position itself to take advantage of ADR services, entered into partnership with Astroscale earlier last year. (See an animation of how that might work.[39]) The magnetic grappling fixtures are being manufactured by Altius Space Machines and are also compatible with a variety of other grapple methods such as “mechanical grasping, Gecko adhesion, electrostatic adhesion and harpoon grappling”.[40] This opens up compatibility with other ADR services.
Returning to the top 200 high-risk list, most of the legacy rocket bodies and defunct satellites have no grappling fixture. In this case, startups like ClearSpace offer multiple robot arms to carefully embrace such uncooperative, tumbling objects. ESA selected ClearSpace from a field of more than a dozen candidates to lead the first mission to remove an ESA-owned item from orbit in 2025.[41] “ClearSpace-1” with its trash treasure will then deorbit and burn up in the atmosphere. An expensive one-off? For this first mission, yes. ClearSpace intends to develop a fleet of on-orbit ADR service vehicles that will remain in space to remove many objects.
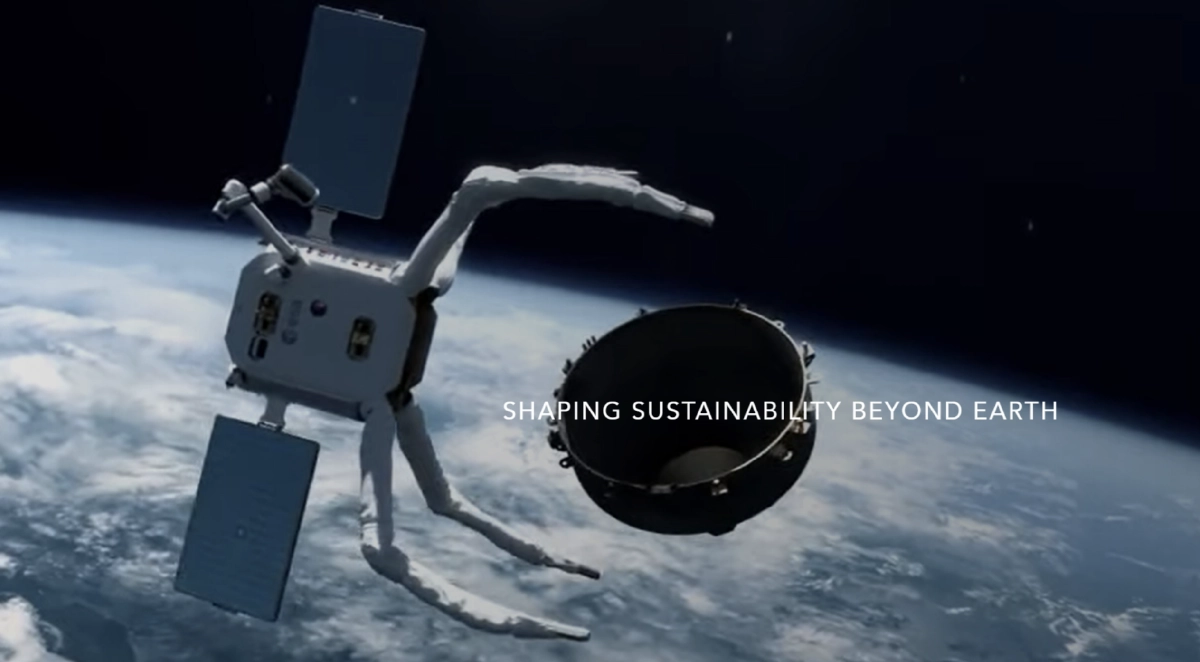
ClearSpace concept uses large robotic arms to encompass objects. Image credit: ClearSpace[42]
Rehabilitation
Not all defunct spacecraft need to be remediated. Some just need to be rehabilitated, with additional propellant, repairs, or even whole-host systems! For example, a spacecraft out of propellant but otherwise still functional could be hosted by another spacecraft. The hosting spacecraft would attach to the client and use its own propulsion and attitude control systems to maintain orbit and pointing.
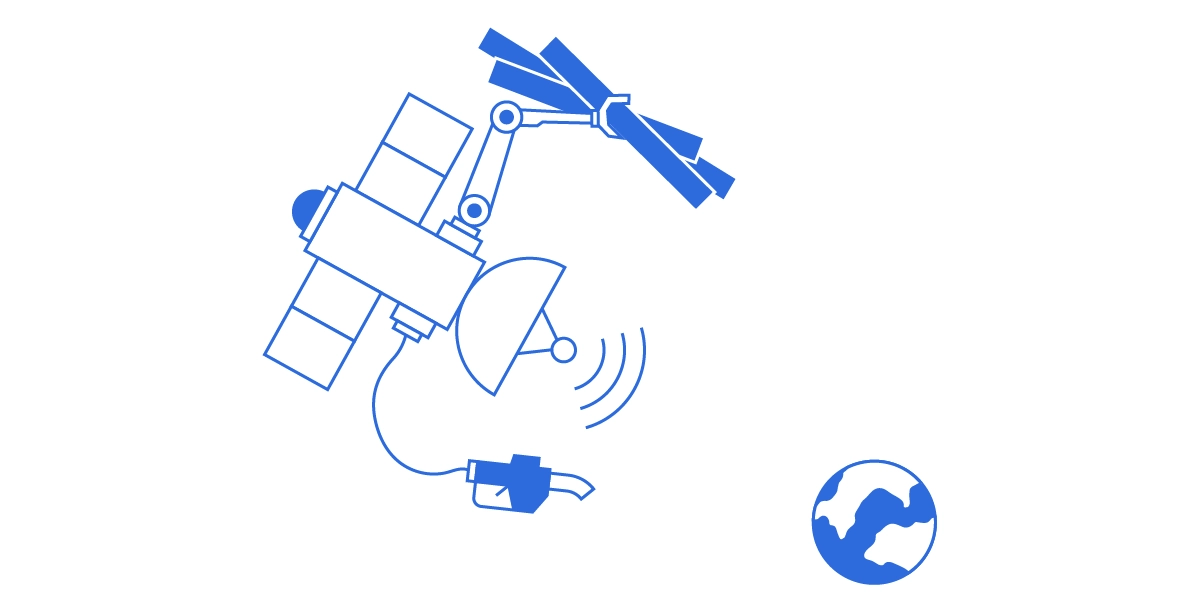
Just last year SpaceLogistics LLC, a wholly-owned subsidiary of Northrop Grumman Corporation (NYSE: NOC), used one of its Mission Extension Vehicles (MEVs) to extend the operational life (and revenue generation) of a several-ton communications satellite – by five years.[43] The intent is that after five years, the MEV will have the capacity to move on to service other satellites. This is the second time an MEV has been used to meaningfully extend the life of a commercial space asset.[44] Even more interesting and challenging, the satellites were designed and deployed before remote on-orbit servicing was possible. SpaceLogistics devised a secure docking method that reaches into the nozzle of the client’s spacecraft engine. SpaceLogistics now has seven missions booked with six customers, through 2025 and into 2026.
Eventually, refueling will be available via startups like OrbitFab. And other on-orbit service companies will make repairs through On-Orbit Servicing, Assembly, and Manufacturing (referred to often as OSAM). Startup Made In Space, acquired in 2020 by Rewire (NYSE: RDW), is participating in a NASA demonstration mission called OSAM-2 to manufacture in space via 3-D printing long-boom solar arrays.[45] Such a service could be used to replace solar arrays degraded over time by orbital debris punctures.
Recycle
Even further into the future, we expect to see satellites recycled rather than just discarded into the atmosphere. Graveyards will be mined for spare parts and materials.
For example, startup CisLunar Industries intends to use materials collected from space debris as feedstock for their patent-pending Micro Space Foundry (MSF). The MSF is based upon electromagnetic levitation furnace technology proven in a terrestrial application. Last year, CisLunar Industries completed a NASA phase 1 SBIR grant to use “multiple, software-controlled electromagnetic induction coils for the generation of gradients and minimums for 3-axis positioning control, movement through the heating elements, and into a desired shape… enabling contactless heating and transport of the metal sample through the entire process.”[46] They have since been awarded a phase 2. CisLunar Industries aims to produce wire, sheet, rods, and tubes in space. Dimensions can exceed those practical for launch from the Earth’s surface. They also are producing metal propellant for their partner Neumann Space’s plasma thrusters. Eventually the MSF will also use feedstock sources from lunar regolith and asteroids!
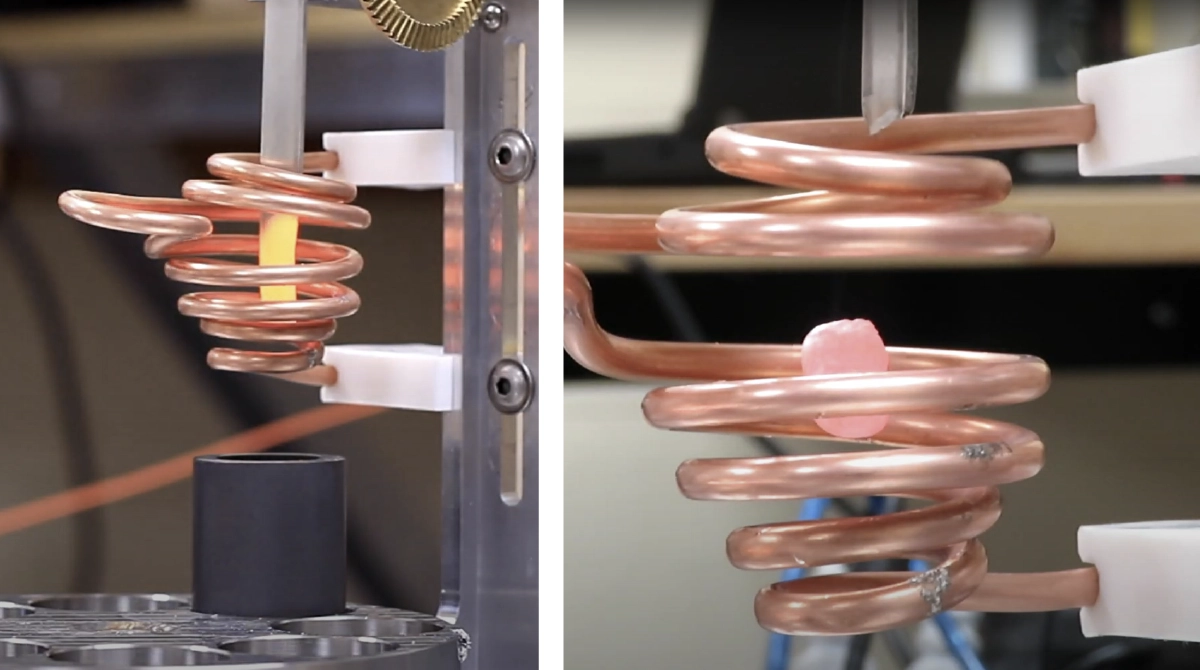
CisLunar Industries demonstration of electromagnetic levitation furnace technology. Image credit: CisLunar Industries[47]
Debris Mitigation
The Inter-Agency Space Debris Coordination Committee (IADC), an international forum of space agencies and authorized governmental entities, publishes the IADC Space Debris Mitigation Guidelines.[48] The members agree, in principle, to mitigating generating debris by:
- Limiting debris released during operations (e.g., exploding separation bolts, peeling coatings)
- Minimizing the potential for on-orbit break-ups (e.g., exploding fuel tanks or batteries)
- Ensuring timely post-mission disposal (e.g., deorbit into the atmosphere or move to a graveyard orbit)
- Preventing on-orbit collisions (e.g., be trackable, be maneuverable)
Various “standards” exist across the (now many) space-faring nations, but the teeth they wield varies. As most are just guidelines, compliance is low, which begs for inexpensive, low-burden solutions, or solutions that significantly lower a company's operating costs. Examples include:
- Designs to reduce debris generated during launch, e.g., from reducing fragmentation caused from explosive bolts used in stage separation to returning whole stages to Earth for reuse.
- Spacecraft materials or shielding that do not produce spall upon impact
- Coatings that do not flake off after thermal cycling in the vacuum of space
- On-board orbital debris detection and maneuverability systems to avoid collisions
- Procedures to “safe” a spacecraft at end-of-mission, like venting residual propellant and pressurized gasses, and discharging batteries
- Adding features that accelerate end-of-mission orbital decay into the atmosphere
For example, decreasing an object's ballistic coefficient (BC) is one way to accelerate orbital decay. A satellite BC can be decreased by deploying large surfaces to increase the cross-sectional area (A) on which the atmospheric drag acts. Deployable devices with large drag coefficients (Cd) and low mass are effective, like sails, inflatable balloons, and tethers that dip into the lower altitudes into the “thicker” atmosphere.
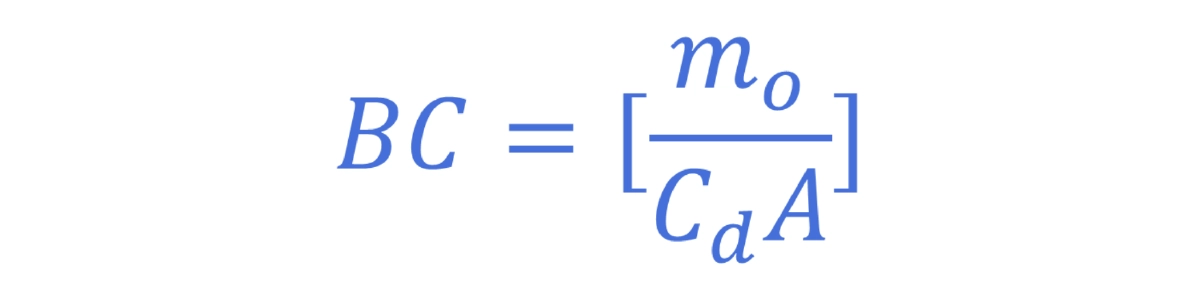
Tethers Unlimited, acquired by AMERGINT Technologies in 2020, offered a Terminator Tape™ Deorbit Module. A thin conductive tape is deployed and induces increased drag on the spacecraft, hastening its orbital decay (at this point) passively. The on-orbit demonstration showed reductions in orbital decay from 10 years to months.[49]
These devices have to be baked into the design of the object before it launches. They also may require communication, computing, power, deploy mechanisms, and/or consumables like inflation gas to be activated. If malfunctions (or a small piece of debris!) renders any of these inoperable, the object’s deorbit can’t be accelerated per plan. Thus responsible operators should incorporate multiple methods, both active and passive.
The various design solutions may originate in-house or they mabe be sourced by suppliers (or a combination of suppliers). No one design mitigation feature is likely VC-backable. However, a startup that incorporates multiple debris mitigation approaches promotes sustainability and lowers their personal risk!
Startup E-Space proposes a constellation of thousands of secure communication satellites. E-Space incorporates both debris mitigation through design as well as passive debris removal.[50] E-Space aims to design its satellites with small cross-sections to decrease the probability of collisions. Satellites will be designed to fail into a high-drag configuration. E-Space eventually intends to evolve the design to trap small debris. Thus, when the satellite eventually becomes inoperable (whether that be due to planned end-of-life or debris-induced damage), the satellite will deorbit with any trapped debris.
Market
Tragedy of the Commons – of the Worst Kind
The challenge is that orbital debris is sort of a “tragedy of the commons.” The “commons” is the environment of the orbital planes in which many inhabit to provide services. There is little incentive for users to remove their decommissioned or defunct assets timely. There is no incentive for users to clean up any generated debris. There is also no entity that is charged to maintain the health of this international environment. Thus the “tragedy” is that this wonderful resource becomes completely consumed (congestion) and, if with uncontrolled debris present, eventually possibly unusable.
Just like other environmentally-related Tragedy of the Commons, space debris accumulates over time and the problem grows worse. Unlike others, space debris could cause a catastrophe in an instant, and as the space infrastructure grows, that catastrophe will not be discriminating. It will take out systems that impact globally, and take decades and billions to rebuild.
Governments Slowly Stepping-Up (A Brief History on Orbital Debris Policy)
The international community has been talking about how to address orbital debris since before the 1990’s. Back then, the focus was mostly on mitigation (i.e., how to prevent it). In 1990, the U.S. Congress under the first Bush administration conducted a study about the causes and distribution of orbiting debris, and examined research and development (R&D) activities for minimizing it.[51] In 1994, the United Nations began work to establish the first space debris mitigation guidelines which were finally published by IADC in 2007. In the meantime, in 2001, the U.S. developed its own guidelines,[37] and in 2004, the Federal Communications Commission followed with rules for satellite operators to show evidence of orbital debris mitigation consideration and planning.[52]
As the orbital debris population was seen to triple by 2010,[53] the Obama administration addressed remediation (i.e., eliminating the debris).[54] Orbital debris not being core to either the Department of Defense (DOD) or NASA missions (and subsequently having no Congressionally allocated budget), progress has been slow. Almost a decade later, the Trump administration issued Space Policy Directive-3 and then updated the National Space Policy. The emphasis on mitigation continued, but any references to removing debris have been, well, removed. To this day, no responsibility for remediation has been assigned by Congress, ideally to the Department of Commerce (DOC).
At this point, the DOD had been tracking objects in space with the Space Surveillance Network and warning of potential collisions. However, the number of countries and commercial companies launching, coupled with the growing debris, was beginning to be overwhelming – and beyond its mission scope. Space Policy Directive-3 directed the Department of Commerce (DOC) to interface with the civil and commercial sectors, and create an “Open Architecture Data Repository.” It has been a little slow to take off, but a prototype, demonstrated in early 2022, has been developed in partnership with the Aerospace Corporation, MITRE, MIT Lincoln Lab, and the University of Texas.[55] The system will provide conjunction warning using government and commercial sources and is expected to be fully operational in 2025.
In the meantime, the FCC updated its rules to be more explicit in how satellite operators address debris mitigation - good to ensure risks are considered with plans in place to mitigate, but still lacking significant incentive for operators to follow through with those plans. Still, the rules are forcing operators to consider sustainability, and the FCC can deny requests if the FCC assesses the plans are not sufficient. (For a very good summary of history and deep dive into the rules, how industry reacted and forward activities, review the “Mitigation of Orbital Debris in the New Space Age” in the federal registry![56])
Early in 2021, the Trump administration released the National Orbital Debris R&D Plan.[57] The plan will be used to guide government funding and to what agencies. The comprehensive plan is broken into three elements: “Limited Debris Generation by Design”, “Track and Characterize Debris”, and “Remediate or Repurpose Debris.” (Given that the National Space Policy does not mention remediation, I was glad to see this third element included!)
Recently the Biden administration solicited comments on the plan. Prime Movers Lab advocated two top priorities within the R&D plan’s element “Remediate or Repurpose Debris”: (a) funding an initial set of remediation demonstrations to establish the first wave of services while immediately removing threats; and (b) performing studies to inform commercial-friendly regulations or collective solutions that will create a market for orbital debris risk management (using any method) while informing investors (who may partner with government) to accelerate deployment.
Of course, entrepreneurs advocated for their tech, for example, to provide on-orbit servicing, better on-orbit maneuverability, in-orbit recycling and spacecraft manufacturing. Space environmentalists, economists, policy, and law experts weighed in. In-space service providers and even private citizens who would benefit from a cleaner and safer environment also participated. A diverse range of opinions and ideas were shared, demonstrating the complexity of the problem – and the magnitude of opportunity! There are a plethora of new entrepreneurs, spawned from the recent massive injection of liquidity into NewSpace and drastic decreases in launch costs. Coupled with government partners and investors, we just may be able to start cleaning up our mess.
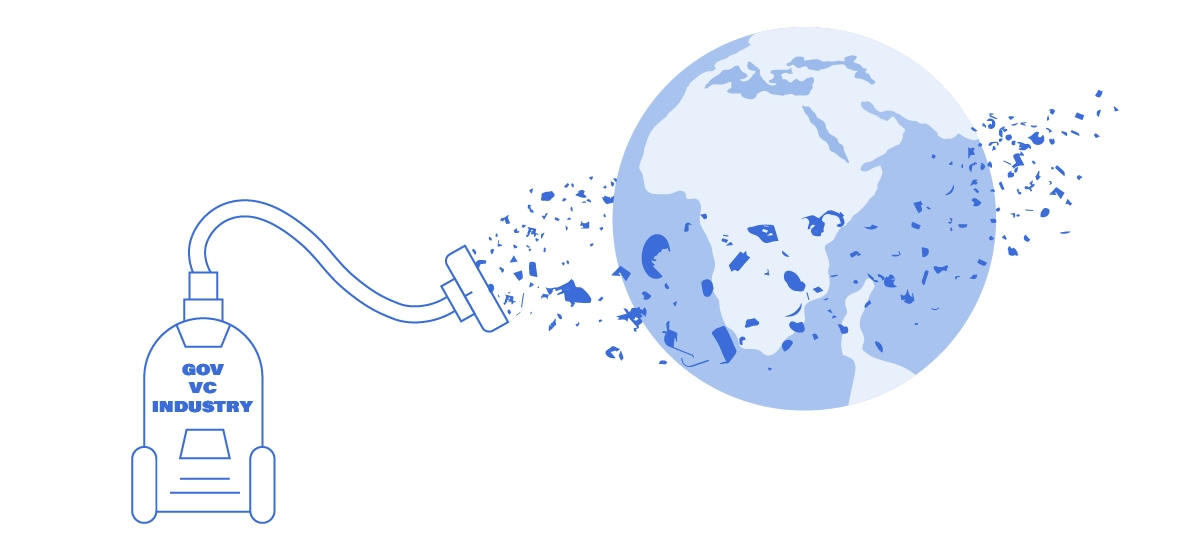
Growing Demand
The global space economy is estimated at $371B.[58] Investments in startup space companies doubled for a record $15B in 2021.[59] Space company entry into the public sector has also been rapidly increasing since 2019, with $4.2B raised through public offerings. The opportunities fueling the next wave are plentiful. We are seeing an increasing number of startups rich in diverse applications and technologies that will build upon each other to provide the infrastructure and lower costs for doing business in space.
So what are these market opportunities? Servicing will be in demand for high-value large GEO satellites. These assets are worth a lot of money. Keeping them on orbit allows for them to generate revenue for longer. Demand for extending the operational life of satellites in geostationary orbit is predicted to create a $3.2 billion cumulative market opportunity within the next decade.[60] GEO satellites also don’t want to turn into a liability when they have exhausted their utility or (worse) become unintentionally inoperative. Space tugs could move them to the graveyard.
On the LEO side, large constellations may be designed to lose a satellite or two (or 10 or 20). Their low cost may also allow for replacement rather than service. But from the debris side, they may choose to equip themselves for ADR. That is being driven by their business and economic decisions to keep their orbital space clutter-free from self-inducing hazards. ADR may be a secondary means to insure deorbit into the atmosphere or hardware that allows a tug to assist.
Over half the respondents at the annual CONFERS 2021 Global Satellite Servicing Forum (GSSF) concluded that space servicing capabilities will immediately contribute the most to achieving sustainability (followed by large-scale science platforms, in-space transportation, exploration, and national security).
So ADR and satellite life extension are the first focus. These capabilities will grow other services. Space tugs will eventually require propellant depots less they become orbital debris themselves. Satellite relocation can then enable customer flexibility. Inspection may be required to assess the state of an inoperative satellite (salvageable or in need of a deorbit assist?). Space tugs could turn into hosts providing functions (like station keeping, power or comm) that maybe were damaged by small debris, a single event upset, or simply end-of-life. On-orbit 3-D printing may provide repaired solar arrays or antenna booms.
And the military could be a big customer, too. The U.S. Space Force Vice Chief of Space Operations, Gen. David Thompson, has been advocating for companies to address the problem, “I’ll pay by the ton if they can remove debris.”[61] Space Force amplified that message last fall as part of a keynote at the Advanced Maui Optical and Space Surveillance Technologies (AMOS) conference, “I think there is a use case for industry to go after that as a service-based opportunity.”[62] Maj General DeAnna M. Burt went on to explain that commercial companies are preferred over military-led efforts to avoid the worry of “dual-use” (where the technology could be classified as useful for weapon applications and slow their adoption). In support of this, Space Force’s business accelerator “Hyperspace Challenge” targets technology areas to effect orbital debris detection, tracking, and removal.
One thing we did learn is that insurance companies are NOT driving market adoption. Insurance is required for launch to ensure no-one in range gets hurt. However, if an operator satisfactorily demonstrates to the FCC that they adhere to the debris generation guidelines, additional insurance for on-orbit operations is not required.[63] Further, insurance won’t pay to remove debris to lower their rates (the cost-to-benefit analysis is unfavorable) nor will they (yet) hold others liable. Liability is just too difficult to prove. The first challenge is to identify WHO is liable. In other words, it is tough to answer, “From where did that fragment originate?!” The second challenge is to show the fragment occurred due to gross negligence. So currently, space is operating like a “no fault” zone. One can still purchase insurance, and some of the higher valued assets (like in GEO) do. Many commercial satellite operators in LEO chose not to buy insurance as their satellite costs are significantly less. For the mega constellations, they have redundancy built in. As I noted, they can lose a satellite or two… or 40 if you are SpaceX![64]
Growing the Market
The following are what we are watching to encourage a growing, sustainable market.
Orbital debris removal demonstrations. Orbital debris will never cease to exist, even with the most well-intentioned designs, and the problem exists now. We have sufficient tracking capability to start remediation and known objects that, if removed, immediately lower the risk. Our recommendation is to fund demonstrations, much like the ESA and the United Kingdom[65] are already doing. In addition, U.S. orbital debris remediation capability protects us against other countries failing to fully enforce mitigation standards and large debris-creating events.
Data to drive commercial-friendly incentives. We are looking for studies to inform smart, agile regulations or collective solutions (agreements between users in the use and sustainable management of space) to enable a market for these remediation services. The intent should be that as remediation technologies are demonstrated, any requirements are put in place to help to close business cases. Doing this in parallel ensures that services exist in time to help entities comply. And only then will meaningful clean-up start to happen. I’ll emphasize again that incentives need to be commercial-friendly. We don’t want to overregulate, but it is naive to rely only on self-regulation. Studies should explore how to make compliance easy and fast, as well as seek international agreement to eliminate unfair competition.
Building out the customer database. We also advocate for determining and publishing ownership of orbital debris. This will provide a resource of potential customers for orbital debris removal services who otherwise could not approach the debris in the first place. Initially, governments may negotiate the removal of high-risk items. Customers may choose to remove their debris to protect trade secrets. Others may be compelled to act responsibly for publicity. The mere fact that ownership is being determined and published may influence operators to be proactive in adopting orbital debris-minimizing tech and better end-of-life mission plans. More likely, when regulations or collective solutions are eventually designed to hold entities accountable, all owners will be required to seek some form of debris risk management or remediation services.
In the long term, the continued identification of owners will feed the market with customers. Developing that database will require continued development of models to discover debris origin as the “easy” pieces are IDed while less characterized and/or smaller pieces require more complex simulation and tracking.
Mitigation through design. We have talked with many companies already successfully pursuing technology that limits debris generation by design. For investment firms, the bottleneck to making a dent in addressing the orbital debris problem is not necessarily funding to create the technology, but rather the market demand to successfully deploy it. So, though we do not advocate novel design approaches as a top investment priority, we do appreciate that government R&D funding serves as a source of non-dilutive funding for operators developing any space system (lowering their financial risk), while incentivizing environmentally-responsible designs that protect the infrastructure.
We caution the government against designing regulations that stipulate design standards as they could unintentionally limit creativity of solutions or (worse) burden service providers. For example, standards that would require active-mission collision avoidance and decision-making could be relatively massive, power consuming, and/or not synergistic with a craft's objectives, limiting capability while increasing costs, etc. It is in an operator's self-interest to protect themselves from a collision. We also caution using standards to force it in a way that stifles innovation or incentivizes industry to move abroad.
In the long term, limiting orbital debris “by design” will start to have meaningful impacts. Industry will be completing design cycles and flight qualifications, and we will start to see deployments of sustainable designs. Specific areas include improving resilience of spacecraft surfaces, shielding and impact resistance, and fragment-reducing technology. Though orbital debris will not be eliminated, we will have tools to make our critical in-space infrastructure more robust to debris as well as less susceptible to originating debris in the first place. With regulation or collective agreements in place to hold ourselves accountable, these design solutions will be sought after.
Landscaping
*Financing data sourced from Pitchbook
Astroscale
Category: large-scale orbital debris mitigation and removal
Differentiator: most mature product on the market w/ current in-space demo mission
Year Founded: 2013
Country: Japan (with subsidiary in US and EU)
Last financing*: $109M Series F in Nov 2021
ClearSpace
Category: large-scale orbital debris removal
Differentiator: capture of high tumbling-rate objects
Year Founded: 2018
Country: Switzerland
Last Financing*: $4.4M Early Stage on $12.5M post in June 2021
Cislunar Industries
Category: large-scale orbital debris recycling
Differentiator: in-space recycling of debris to make metal propellant rods for plasma thrusters
Year Founded: 2017
Country: USA
Last Financing: n/a (bootstrapped, SBIR grants)
D-Orbit
Category: large-scale orbital debris mitigation
Differentiator: grapple bar for satellite servicing/ deorbit and D3 (smart, propulsive D-Orbit Decommissioning Device)
Year Founded: 2011
Country: Italy
Last Financing*: announced SPAC in Jan 2022; $1.3B post in April 2022
EOS
Category: small debris remediation
Differentiator: laser to change local drag of microparticles for de-orbit
Year Founded: 1983
Country: Australia
Last Financing: n/a [publicly traded company (ASX: EOS)]
ExoAnalytic Solutions
Category: Space Traffic Management
Differentiator: partnered with NorthStar on collision avoidance
Year Founded: 2008
Country: USA
Last Financing: n/a (no venture financings/bootstrapped, DOD grant)
Kayhan Space
Category: Space Traffic Management
Differentiator: Autonomous avoidance navigation algorithm
Year Founded: 2019
Country: USA
Last Financing*: $4.4M Seed on $14.5M post in Dec 2021
Kurs Orbital
Category: large-scale orbital debris removal
Differentiator: leveraging decades of Ukranian Space Agency rendezvous, proximity operations and docking (RPOD) technology for their system
Year Founded: 2020
Country: Ukraine
Last Financing*: $6.5M angel invest in March 2021
LaunchSpace Technologies
Category: small orbital debris removal
Differentiator: large multi-layered aerogel catcher’s mitt
Year Founded: 2020
Country: USA
Last Financing*: $250K crowdfunding Sept 2021
LeoLabs
Category: Space Situational Awareness/ Debris Tracking
Differentiator: terrestrial network of cost-effective phased-array radars with global coverage for orbital debris tracking
Year Founded: 2016
Country: USA
Last Financing*: $65M Series B on $380M post in June 2021
NorthStar
Category: Space Situational Awareness/ Debris Tracking
Differentiator: on-orbit optical satellite constellation
Year Founded: 2011
Country: Canada
Last Financing*: $45M private equity growth round
Numerica
Category: Asset Monitoring/ Debris Tracking
Differentiator: telescope network for asset tracking & monitoring
Year Founded: 1996
Country: USA
Last Financing: n/a (no venture financings/bootstrapped)
Orbion Space Technology
Category: orbital debris mitigation
Differentiator: high-impulse avoidance maneuver propulsion
Year Founded: 2016
Country: USA
Last Financing*: $20M Series B on $112M post in Oct 2021
Portal Space Systems
Category: large-scale orbital debris removal
Differentiator: propulsion system that allows high delta-V maneuvers from MEO
Year Founded: 2021
Country: USA
Last Financing: $500K pre-seed
Privateer Space
Category: Space Situational Awareness/ Debris Tracking
Differentiator: space situational awareness software and debris catalog including debris characterization information
Year Founded: 2021
Country: USA
Last Financing: unclear sized (multiple different data points - $5M on Crunchbase, $12M on Pitchbook) Seed round announced in Dec 2021
SCOUT
Category: Asset Monitoring/ Debris Tracking
Differentiator: collision warning and RPOD optics, on-orbit SSA, focused on DOD and IC
Year Founded: 2019
Country: USA
Last Financing*: undisclosed size Seed round raised in March 2022
Slingshot Aerospace
Category: Space Traffic Management
Differentiator: facilitating easy international traffic management coordination, space weather modeling
Year Founded: 2016
Country: USA
Last Financing*: $25M Series A in March 2022 for undisclosed valuation
Starfish Space
Category: large-scale orbital debris removal
Differentiator: autonomous RPOD software that can use electric propulsion
Year Founded: 2018
Country: USA
Last Financing*: $8M Seed on $29M post in Sept 2021
Tethers Unlimited
Category: debris mitigation
Differentiator: drag increasing deployable tether
Year Founded: 1994
Country: USA
Last Financing: n/a (acquired in 2020 by privately held AMERGINT Technologies)
Turion Space
Category: large-scale orbital debris removal
Differentiator: high delta-V multi-mission vehicle
Year Founded: 2020
Country: USA
Last Financing*: $4.9M Seed in Nov 2021
Vyoma
Category: Space Situational Awareness/ Debris Tracking
Differentiator: on-orbit optical satellites with edge compute visual data processing and machine learning algorithm for debris tracking reduces total number of optical satellites needed in constellation
Year Founded: 2020
Country: Germany
Last Financing: currently raising Series A round (as of April 2022)
Conclusions
We need a catalyst – other than a catastrophic event – to motivate the market demand for debris management. Funded missions and commercially-friendly incentives would make investors more confident in the business case of orbital debris-focused companies and funnel more dollars to them sooner, accelerating their ability to act. We recognize cleaning up orbital debris is needed now, but we have a fiduciary responsibility to our investors – will funding these critical startups return funds timely enough?
We have seen a number of companies that can be ready to demonstrate services in the next 2-4 years. So for the near term, the fastest path to impacting the situation is actually removing debris. Not only do they remove debris, but the removal of key objects can lower the risk of more creation. Demonstrated in-space systems are then also posed to provide other on-orbit services. Regulations and/or collective agreements should be made by Biden’s administration to not lose momentum through an election cycle. The timing of orbital debris mitigation demonstration missions and updated commercial-friendly policy could be the catalyst we need.
Acknowledgements
We are grateful to many who provided insight and shared their experiences:
Anousheh Ansari of XPRIZE Foundation,
Brian Weeden of Secure World Foundation,
Carolyn Belle of Astroscale,
Charles Lee Mudd Jr. of Mudd Law,
Chris Kunstadter of AXA XL space insurance,
Ezinne Uzo-Okoro of the White House Office of Science and Technology Policy,
Mike Beavin of Boundary Stone Partners,
Moriba Jah space environmentalist of The University of Texas,
Sven Eenmaa of ISS National Lab,
and the many inspiring “Prime Movers”, the founders and entrepreneurs who are on a mission to transform billions of lives.
Special thanks goes to Liz Stein for engaging in many thought-provoking discussions and creating the Landscaping.
References
[1] Satellites to be Built & Launched, Euroconsult, December 2021
[2] Space Debris by the Numbers, The European Space Agency, March 2022
[3] David, Leonard, Effects of Worst Satellite Breakups in History Still Felt Today, Space.com, January 28, 2013
[4] Letzter, Rafi, Two Satellites Might Collide at 32,000 Mph Over the Arctic Today, Live Science, April 9, 2021
[5] Raju, Nivedita, Russia’s Anti-satellite Test Should Lead to a Multilateral Ban, Stockholm International, Peace Research Institute, December 7, 2021
[6] Distribution of Space Debris in Orbit Around Earth, The European Space Agency, January 2019
[7] Rigby, Mark and Carter, Brad, A Chunk of a Chinese Satellite Almost Hit the International Space Station. They Dodged It — But the Space Junk Problem Is Getting Worse, Space.com, November 15, 2021
[8] International Space Station Forced to Dodge Debris, Russian Space Agency Says, Sky News, December 3, 2021
[9] NASA Administrator Statement on Russian ASAT Test, NASA Release 21-156, November 15, 2021
[10] Zafar, Ramish, NASA Asserts Starlink Doubts Are Not Meant For FCC License Rejection, Wccftech, March 21, 2022
[11] Jones, Andrew, Breakup of China’s Yunhai-1 (02) Satellite Linked to Space Debris Collision, Space News, January 11, 2022
[12] Henry, Caleb, Intelsat Still Searching for Cause of IS-29e Loss, Replacement Satellite TBD, Space News, April 30, 2019
[13] Greenfieldboyce, Nell, Where Falling Satellite Lands Is Anyone's Guess, NPR, September 21, 2011
[14] Roulette, Joey, SpaceX Rocket Debris Lands on Man’s Farm in Washington, The Verge, April 2, 2021
[15] Foust, Jeff, House Bill Would Designate Space as Critical Infrastructure, Space News, Jun 4, 2021
[16] Image of ODTK Sensor fusion, Orbit Determination Tool Kit (ODTK), AGI, accessed March 22, 2022
[17] Abraham, Andrew, GPS Transponders for Space Traffic Management, The Aerospace Corporation, April 2018
[18] Pultarova, Tereza, SpaceX Starlink Satellites Responsible for Over Half of Close Encounters in Orbit, Scientist Says, Space.com, August 18, 2021
[19] Stevenson, et al., Identifying the Statistically-Most-Concerning Conjunctions in LEO, Advanced Maui Optical and Space Surveillance Technologies Conference (AMOS), Maui, Hawai’i, USA, August 2021
[20] Maidenberg, Micah, SpaceX’s Plans to Send Thousands More Satellites Into Orbit Worry NASA, The Wall Street Journal, February 15, 2022
[21] What Is the Smallest Size Satellite LeoLabs Can Track?, LeoLabs, accessed 13 March 2022
[22] Sgobba, Tommaso, and Allahdadi, Firooz, Chapter 8 - Orbital Operations Safety, Safety Design for Space Operations, Elsevier, 2013
[23] Kirkpatrick, Keith, A Traffic Cop for Low Earth Orbit, Communications of the ACM, May 2021, Vol. 64 No. 5, Pages 15-17
[24] Global Phase-Array Radar Network, LeoLabs, accessed March 13, 2022
[25] Werner, Debra, LeoLabs Raises $65 Million in Series B Funding Round, Space News, June 3, 2021
[26] Harrison, Ruth, LeoLabs to Build New Space Situational Awareness Radar in WA, Space Australia News, October 21, 2021
[27] SCOUT Is on Orbit!, SCOUT, July 1, 2021, accessed on 13 March 2022
[28] Rainbow, Jason, NorthStar SSA and Earth Intelligence Constellation Gets Key Government Approval, Space News, August 24, 2021
[29] Foust, Jeff, Spire to Build Space Situational Awareness Satellites for NorthStar, Space News, March 16, 2022
[30] SES Partners with NorthStar to tackle Space Sustainability Challenges, NorthStar Earth & Space, March 31, 2022
[31] Image of Continuous Custody, Congestion in Space is a Clear and Present Danger to All Satellites, NorthStar Earth & Space, accessed on March 13, 2022
[32] Foust, Jeff, Privateer Unveils Technology for Improved Tracking of Space Objects, Space News, March 4, 2022
[33] Werner, Debra, Skycorp to Test “USB for Space” Cable Outside ISS, Space News, March 2, 2022
[34] Debris Remediation, Orbital Debris Program Office, NASA, accessed on March 13, 2022
[35] Treaty on the Principles Governing the Activities of States in the Exploration and Use of Outer Space, including the Moon and Other Celestial Bodies, United Nations, New York
[36] McKnight, et al, Identifying the 50 Statistically Most-Concerning Derelict Objects in LEO, 71st International Astronautical Congress (IAC) – The CyberSpace Edition, Dubai, UAE, October 2020
[37] U.S. Government Orbital Debris Mitigation Standard Practices, Washington, D.C. 2001, updated November 2019
[38] Pultarova, Tereza, Astroscale’s Space Junk Removal Satellite Aces 1st Orbital Test, Space.com, August 27, 2021
[39] Grush, Loren, Watch Internet-From-Space Provider OneWeb Launch Its Biggest Batch of Satellites Yet, The Verge, February 6, 2020
[40] Altius Space Machines’ DogTags™ Grapple Fixtures Launch Aboard OneWeb Satellites, satnews, January 14, 2021
[41] Mathewson, Samantha, ESA Partners With Startup to Launch First Debris Removal Mission in 2025, Space.com, May 16, 2021
[42] Shaping Sustainability Beyond Earth, ClearSpace Today, accessed March 13, 2022
[43] Coldewey, Devin, Another Milestone for In-Space Servicing as Northrop Grumman Gives Aging Satellite New Life, TechCrunch, April 12, 2021
[44] MEV-1 and MEV-2, eoPortal Directory, Earth Sharing Observation Resources, accessed March 31, 2022
[45] STMD: Tech Demo Missions, On-Orbit Servicing, Assembly, and Manufacturing 2 (OSAM-2), NASA, accessed March 13, 2022
[46] NASA SBIR 2021-I Solicitation Proposal Summary, Proposal Number 21-1-Z3.003-1339, NASA, April 6, 2021
[47] CisLunar Industries 2021-Q3 Intro, YouTube, September 29, 2021, accessed 13 March 2022
[48] IADC Space Debris Mitigation Guidelines, Inter-Agency Space Debris Coordination Committee, 2021, Technical Report, IADC-02-01 Rev. 3
[49] Werner, Debra, Electrodynamic Tethers Speed up Satellite Reentry Timelines, Space News, August 12, 2021
[50] E-Space Announces Largest Space Seed Round Ever to Democratize Space with Sustainable Satellites and Reduce Orbital Debris, Cision PR Newswire, February 7, 2022
[51] Orbiting Debris: A Space Environmental Problem-Background Paper, U.S. Congress, Office of Technology Assessment, OTA-BP-ISC-72 (Washington, DC: U.S. Government Printing Office, September 1990)
[52] Mitigation of Orbital Debris, FCC, 69 FR 54581 (09/09/2004), Jun 21, 2004
[53] About Space Debris, The European Space Agency, accessed on March 22, 2022
[54] National Space Policy of the United States of America, NASA, June 28, 2010
[55] Hitchens, Theresa, NOAA Plans ‘Initial’ Civil Alternate to DOD Space Tracking System by 2024: Senior Official, Breaking Defense, February 11, 2022
[56] Mitigation of Orbital Debris in the New Space Age, Federal Register, August 25, 2020
[57] National Orbital Debris Research and Development Plan, United States Government, January 2021
[58] 2021 State of the Satellite Industry Report (SSIR), BryceTech, Satellite Industry Association (SIA), June 2021
[59] 2022 Startup Space Report, BryceTech, April 2022
[60] Bokhari, Syed Hussain Haider, In-Orbit Servicing: Stepping Up To The Challenge?, NSR, February 17, 2021
[61] Erwin, Sandra, U.S. Space Force Would Support Commercial Services to Remove Orbital Debris, Space News, March 16, 2021
[62] Foust, Jeff, Space Force Backs Development of Commercial Orbital Debris Removal Systems, Space News, September 15, 2021
[63] Sims, Eleni M., and Braun, Barbara M, Navigating the Policy Compliance Roadmap for Small Satellites, The Aerospace Corporation, November 2017
[64] Malik, Tariq, SpaceX Says a Geomagnetic Storm Just Doomed 40 Starlink Internet Satellites, Space.com, February 8, 2022
[65] UK Working With Global Partners to Clear Up Dangerous Space Debris, UK Space Agency, October 26, 2021