Additive Manufacturing (AM)
Prime Movers Lab
January 2022
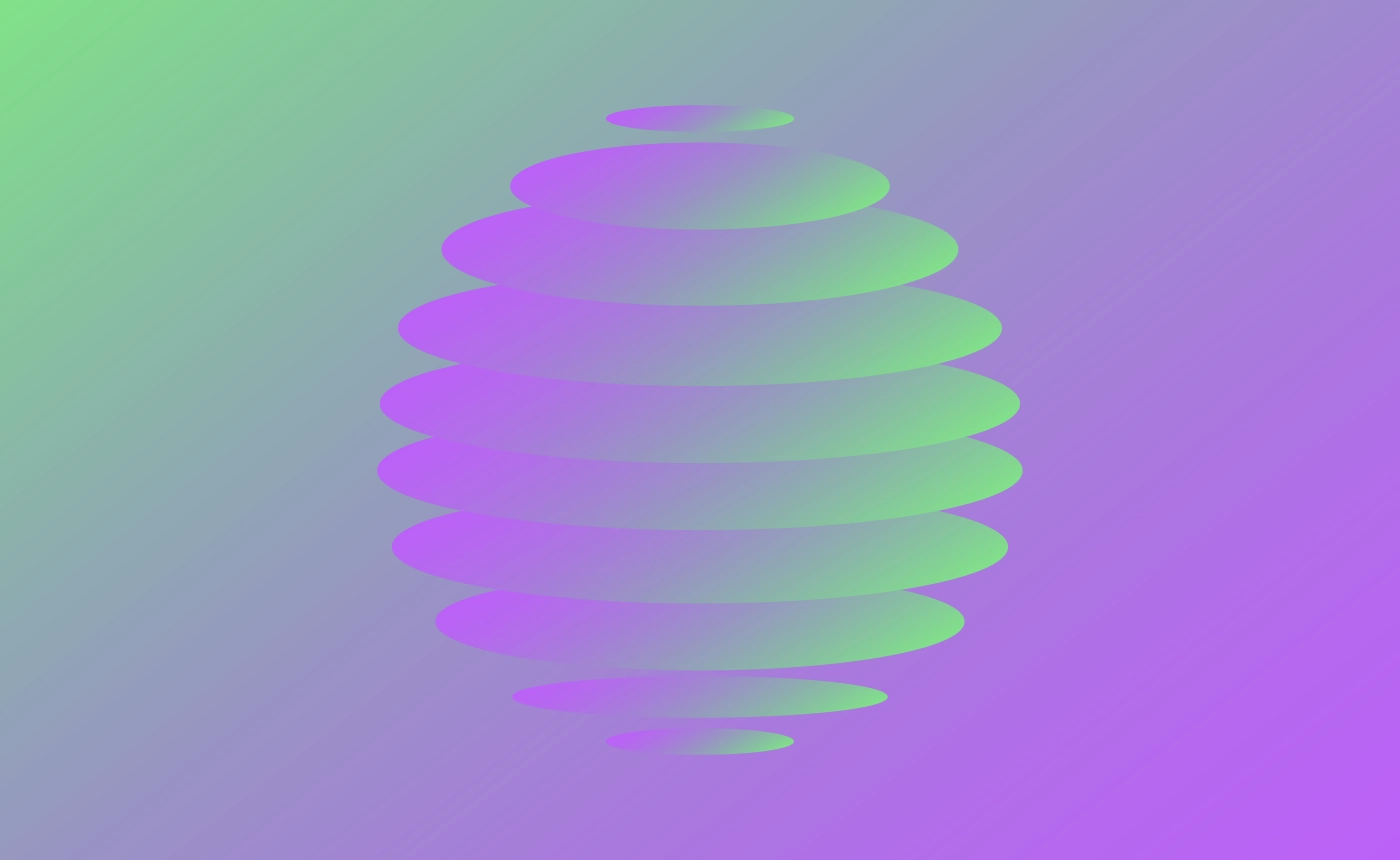
Summary
Additive manufacturing (AM) is on it’s third wave of adoption. Like many other technologies, the latest advances in AM reflect the lessons learned from previous attempts. Those insights have helped AM adapt to a few key deployments that will make it’s adoption successful. Key areas include finding product niches where other technologies, like injection molding or advanced machine and assembly, are sub optimized due to inherent features in both the process and the product. Another area advancement aiding in the adoption of AM is the increasing availability of AM-specific design capabilities, standards, and material that further optimize the output of the AM process including software advancements that simulate and improve initial designs. Overall, a key part of the adoption of AM now is using AM as part of a full stack solution that includes other robotic and automation deployments.
Introduction: How Additive Manufacturing currently fits into manufacturing
U.S. manufacturing output has contributed about $2.3 trillion or 11 percent of US GDP (of the ~$13-14 trillion globally) to the U.S. economy each of the past few years, represented by approximately 250,000 firms engaging in manufacturing activities. Also, while the percentage of manufacturing in the U.S. GDP has decreased over the past few decades, manufacturing has become more capital- and knowledge-intensive rather than heavily industry-based and labor-intensive as it was in the past. Additive Manufacturing (AM) is a relatively small but rapidly growing sub-sector of this transition. According to NIST, AM deployment is one of the top priorities for both large and small US Manufacturers.
AM or 3D printing (overlapping but fringe case definitions differ but from herein referred to as AM) is a broad set of tools being developed for almost every industry. From biological to clothing, and aerospace to art. AM has been around for over 40 years and started commercialization in the early 1990s. It gained early traction in the 2000’s as its potential was realized to minimize waste and decrease the number of components. In the early 2010’s, AM started on its current explosive growth pattern, with the number of printer manufacturers increasing and the availability of AM printable materials coming online. In 2019, the global AM market size was USD ~$6 billion, and it is expected to reach USD ~$40-50 billion by the end of 2026, with a CAGR of 30 percent during the forecast period 2021-2026. Some analysts forecast that the AM market will grow to ~$235 billion by 2030. The key growth drivers for AM are precision, limited material waste, reduced time to market for new products.
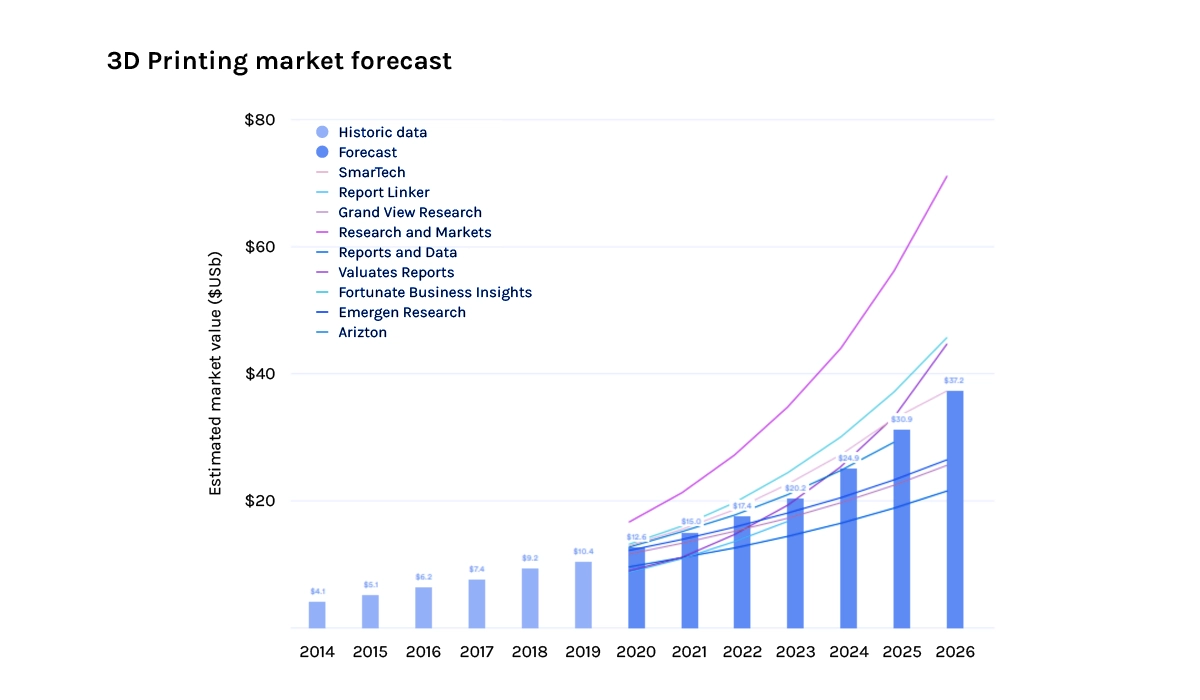
Source: 3dprintingindustry.com
Where Additive Manufacturing is heading
Long-term market consolidation in the printer market is already underway. In just 2021, we have already seen large acquisitions from major AM manufacturers such as Nano Dimension and Desktop Metal. With over 200 printer manufacturers competing across the seven technology types, the differentiation is becoming less pronounced and the need to help integrate these machines will become more relevant. Many new printer companies are creating new terminologies for their printing processes, but they are mainly subtle variations of the existing processes.
Advances in materials will be a crucial driver, enabler of AM, and the background driver in recyclability of used and unused materials. Companies like 6K are driving this field forward and the potential for a full-stack circular manufacturing solution. Also, the materials, ranging from polymers to metals to ceramics to specialized bio and construction materials, are becoming more differentiated from their typical non-AM materials counterparts.
Highly specialized, or difficult-to-service, end markets is another potential application that AM can disrupt. An example is the use of AM in bio printing. The key here will be for a company that has a portfolio of products, potentially using critical platforms to deploy their technology across a number of product offerings.
A potential VC investment into AM would be a company focused on integrated tech plans, including AM in an opportune target end market. AM will be an important part of larger manufacturing trends, including localizing and reshoring manufacturing where products are produced closer to the final assembly facilities and the end customer,for reduced costs, and shortened lead times. According to BofA, “ Companies in 50 percent of global sectors in North America intend to ‘reshore’.”
Another key part of AM trends is the smart factory, that reduces labor content and automates vast portions of the manufacturing process.
AM “myths” to watch out for include:
- AM will wholesale, replacing conventional manufacturing
- Complexity in AM is free
- AM as a “canned” off the shelf process
- AM produces parts inexpensively
- AM parts are inferior to conventional made parts
- Every household will have a 3D printer to service all needs
The layers of Additive Manufacturing

AM consists of six key segments: design for AM, material development, equipment/technology selection, AM part production, post-processing, and additional services.
First layer: AM Design and Engineering
There are two aspects to the design and engineering phase of AM.
The first part of this segment of the AM value chain is driven by software development that focuses on aiding and accelerating “Design for Additive Manufacturing” (DfAM). DfAM has lagged the other areas of the AM value chain as AM has been highly focused on the process. For example, many aerospace components were not DfAM and the full advantages of DfAM, like organic stress paths, weren't being applied so AM parts were a costly replacement for legacy parts. Once an AM-specific design is applied to a part, it often can become lighter., For example when the internal structure more organically follows the stress loads that are exerted on the part.
The second part of this phase is prototyping, the most common use of AM, to provide a quick check for fit and function. It is often cheaper than sourcing prototypes through the conventional supply chain. There are many advances going on in this area including material standards (including temperature tolerances, flexibility, compression strength) and AMspecific design packages that will help AM become more competitive with other forms of manufacturing.
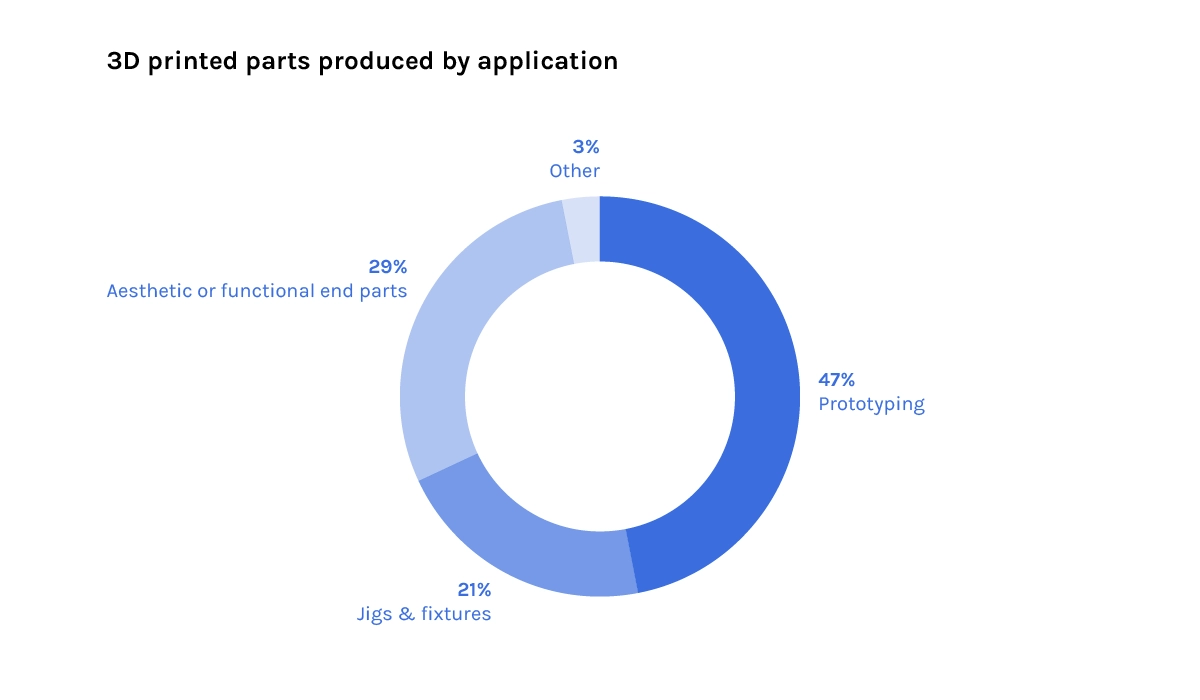
Prototyping isn't growing as fast as other parts of the AM industry, but is still expected to remain a key driver for the next decade. Also, AM-specific software is gaining traction; and optimization for AM- and full-life simulations are in their infancy. Another factor is education and training, and as younger generations are exposed to the AM mindset, DfAM will become more intuitive.
Second Layer: AM Materials
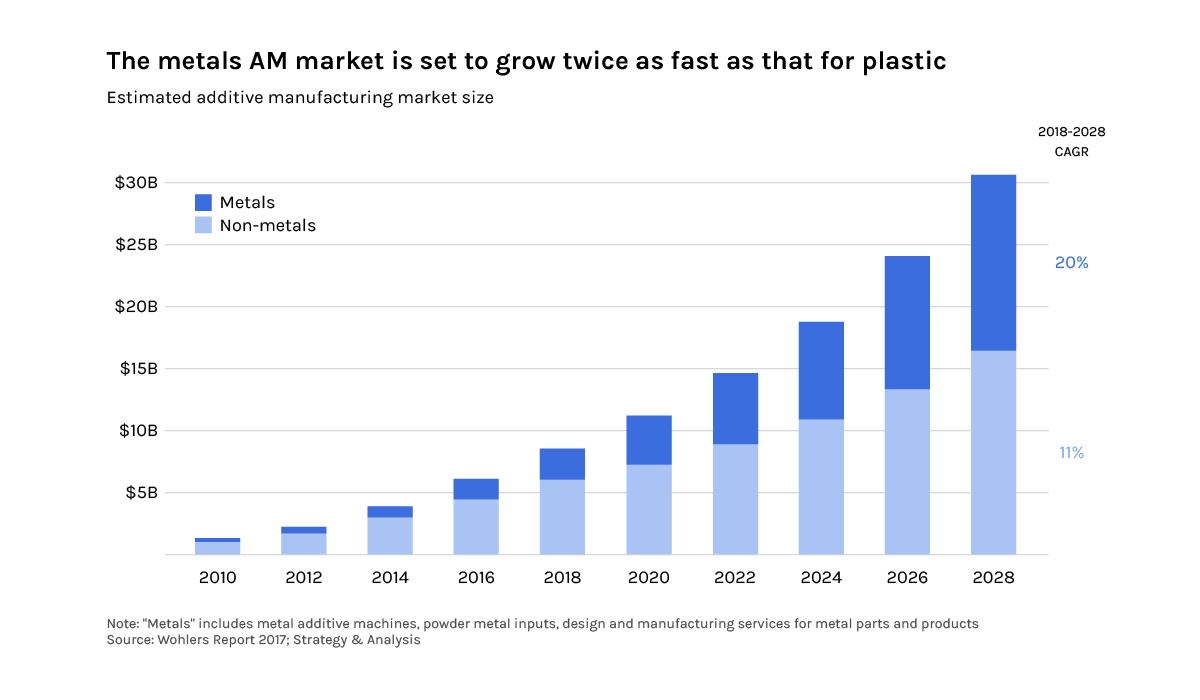
AM materials are divided into three market segments: Polymers, Metals, and other.
Polymers
Polymers are currently the most popular material for 3D printing, including LDPE, HDPE, acrylics, HIPS and PEEK.
Key Trends in Polymer ($147M in 2019 -> $600M by 2027):
- Development of Particle- and Fiber-Reinforced Polymer Composites for added strength; also Thermosets like PEEK
- Nanocomposites development continues for bio and electrical uses
- Increased focus on circular manufacturing and recycling end of life materials
- Material availability and scalability
Key Trends in Metal AM (~$50M in 2019 -> ~$300M by 2027):
- Development of qualification and certification methods is accelerating
- Structure optimization
- Development of more “exotic materials” is gaining traction; eg. copper is hard to work with using legacy processes
- Fastest growing market for AM is for metal powder
Other AM materials
Ceramics are used to print everything from jewelry to bone implants. Ceramics is a growing AM application where many of the large AM printer companies have a ceramic-capable printer.
Concrete is another rapidly growing application ($20M in 2020 to $450M by 2027 with projections in this market varying significantly) with much of the growth coming from Asia and Europe.
Bioprinting includes a vast range of materials from bio-inks for tissue engineering (3D bioprinting market size was valued at USD 1.4 billion in 2020 and is expected to expand at a compound annual growth rate (CAGR) of 15.8 percent from 2021 to 2028).
Key Trends in Other AM Materials:
- Disruptive automation that includes AM
- Advances in hard to work with materials
- Highly specialized, high-value parts, particularly in low-rate production environments like aerospace, and highly customized spaces like medical devices and high-end cars
Third Layer: Additive Equipment
There are more than 200 printer manufacturers, including 60+ with multiple offerings and over 100 in the startup phase. These manufacturers cover a range of technologies and many use their own terminology for similar products. Some of the key advancements in the equipment industry include the development of high resolutions, faster machines with multiple heads to increase throughput, integrated systems, nanotechnologies, and industry-specific technologies.
There has also been a consolidation of printer manufacturers with Stratasys, 3D Systems, Desktop Metal (also going public), and a few others leading the way on acquisitions as they grow their IP and technology portfolios and increase their offering across a platform of solutions. This consolidation will help standardize the industry and bring together smaller startups to take their technologies to a global stage.
Fourth Layer: Additive Manufacturing Technologies
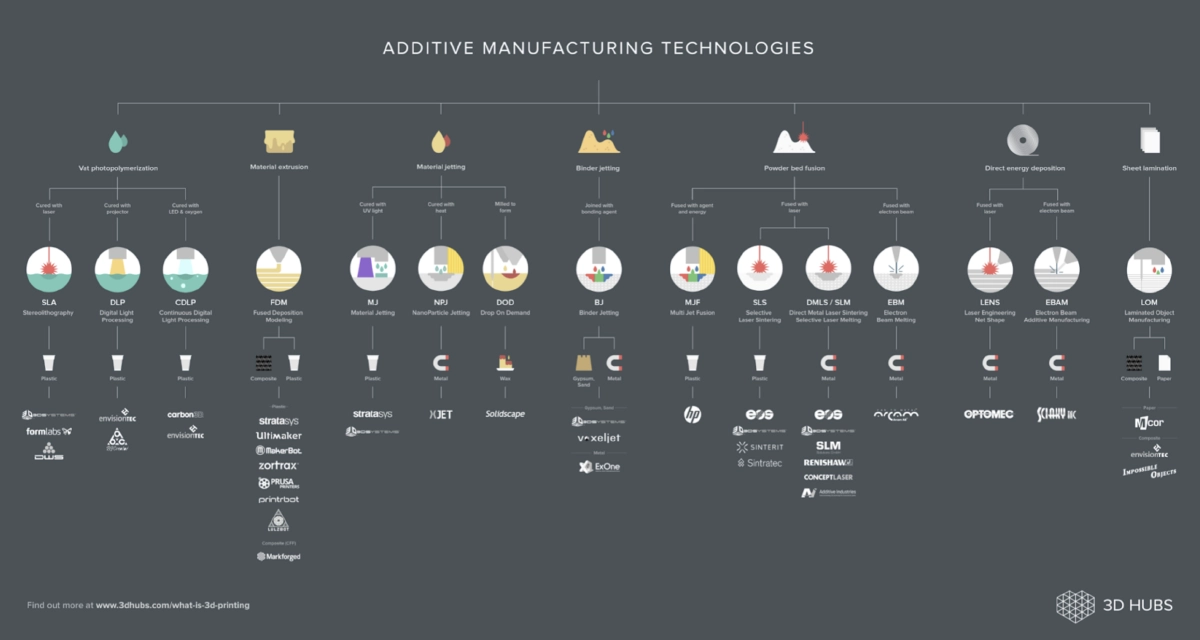
Source: 3D Hubs
The AM market processing technologies:
Vat photopolymerization
Vat photopolymerization, including stereolithography (SLA) and direct light processing (DLP), is a group of processes that use a vat of liquid photopolymer resin that is cured in a specific shape, only layer at a time. The process uses UV light source, mainly from a projection or laser vector scanning, reacting with a resin in the liquid that bonds together and forms a layer of the final part. As each layer is cured, the light source moves to enable the subsequent layers to be built up. Once the part is complete, all remaining resin is drained. These processes include stereolithography (SLA) and direct light processing (DLP).
Vat photopolymerization processes are used in a wide range of industries, from flexible electronics. to orthotics and medical devices. to sports equipment and advanced composites. The future of these processes lie in advancements in material chemistry and resin systems including electroactive polymers, artificial organs and muscles, and superhydrophobic materials.
Material Extrusion
Material Extrusion is the process of pushing or drawing material,through a nozzle with a specifically designed cross-section. The material is heated as it passes through the nozzle then layered horizontally as the nozzle moves vertically and the platform, or build stage, moves. Material extrusion is the most common form of AM, particularly for hobbyists and others looking for an inexpensive AM build. It is used to replace injection molding processed parts with better control over the build up for parts with more advanced structures. The key to further developments in material extrusion range from further advancement of the extruder itself, to increase speed and accuracy and within the types of materials being extruded including bioavailable materials for food and medical products and advanced construction materials.
Material Jetting
In a process inspired by and similar to an inkjet printer (2D of course), material jetting (MJ) sprays or jets material, either in droplets on demand or continuously, on to a build stage, layer by layer. The material is cured using UV or heat, one layer at a time. While it is currently limited to specific polymers, waxes and a few other materialst, MJ is considered to be one of the fastest and most precise methods of AM. MJ is also advantageous in that it can do multi-part builds in parallel. It also produces a good surface finish and is one of the most advanced AM processes in the use of multicolor printing. MJ is limited to non-structural parts, but this technology has good potential in “nano” builds and high specificity designs.
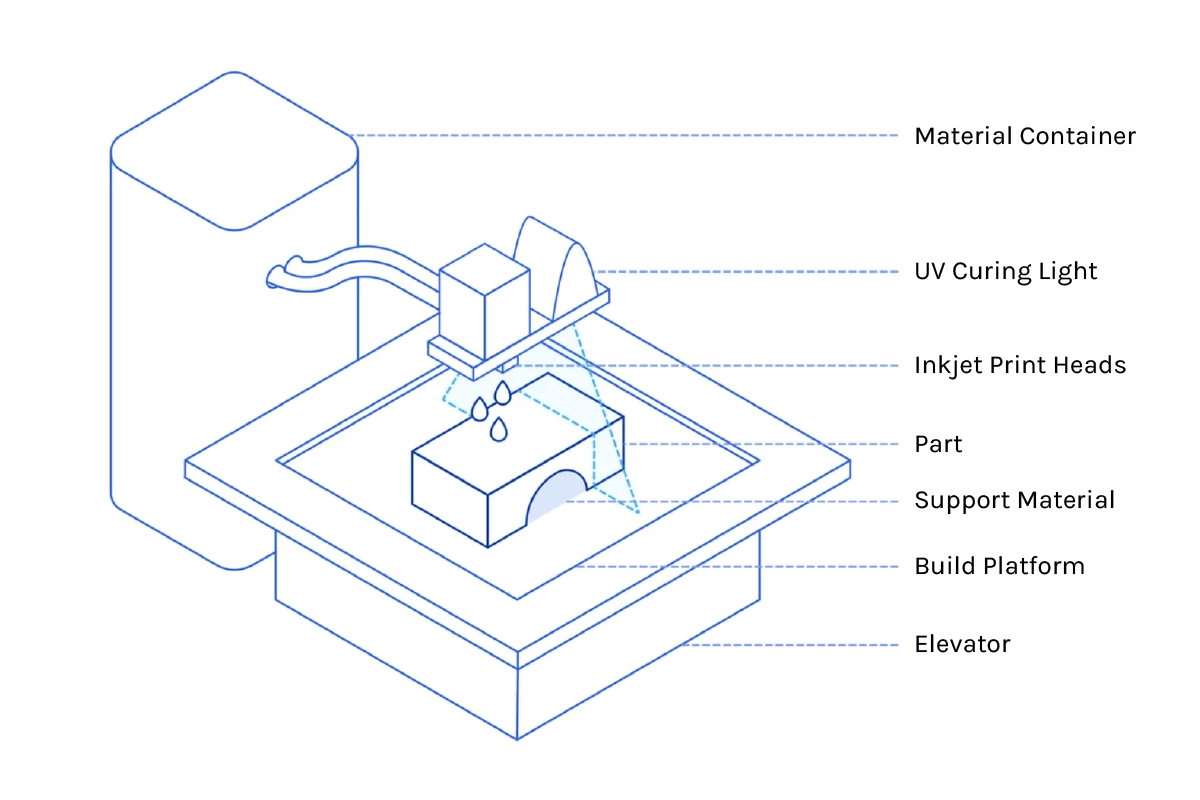
Binder Jetting
In Binder Jetting, the head moves along the x y plane, jetting binding liquid onto a powder-bed build stage. As each layer is finished, the build stage lowers and a thin layer of powder is deposited on the part, before the head sprays the next layer. This process is repeated until the part is complete. Binder jetting differs from other AM processes in that it does not use heat during the layering progress. This makes binder jetting one of the quicker processes and the part dimensions more accurate, though there can be significant time added in post processing . Binder jetting also does not require sacrificial material or structures as it is supported by the surrounding non-binded powder.
Binder jetting is one of the more flexible AM processes. It can be used for a variety of materials including ceramics, sand, metals and alloys. It is often used to create large parts or casting cores and molds and the structural quality is consistent with metal injection molding. With this flexibility, and the higher speeds it can achieve, the process will be important to unlocking shorter supply chains as advances in the sintering and binding process reduce the overall build cost.
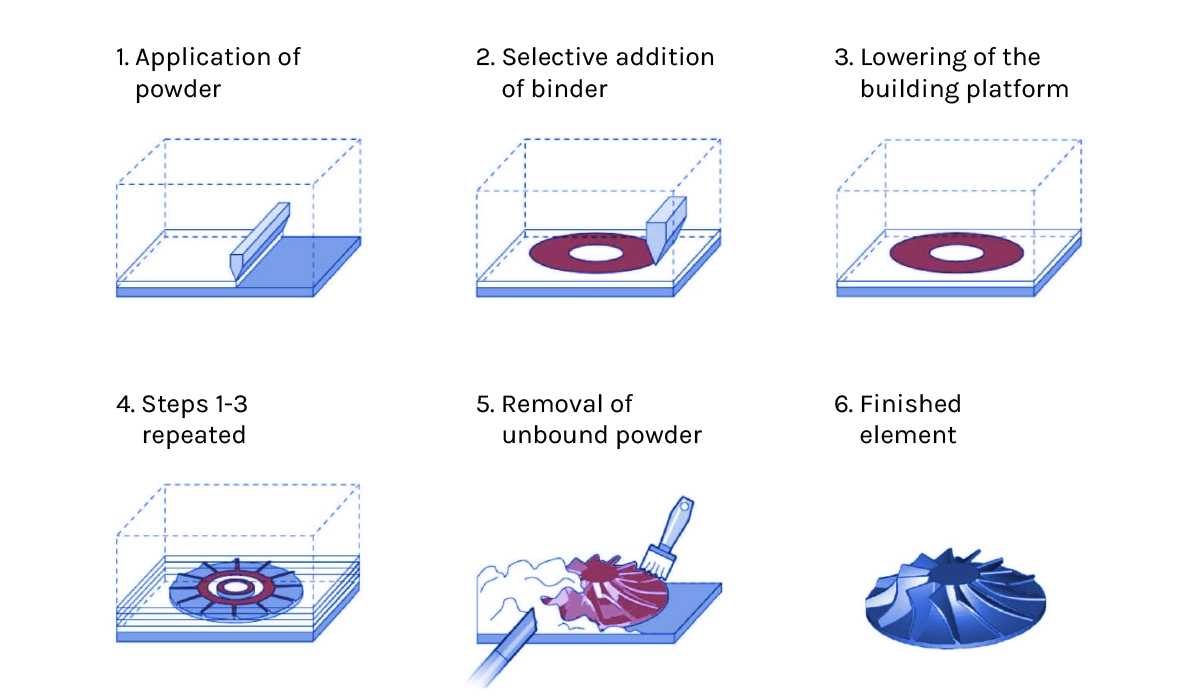
Source: Engineering Product Design
Powder bed fusion
Powder bed fusion including Direct metal laser sintering, Selective laser sintering, Multi Jet Fusion, Electron beam melting, Selective laser melting and Selective heat sintering, is the use of a laser or electron beam to melt the powdered material, fusing it to create a solid object. It is one of the original AM technologies.
The growth in PBF is being driven by advances in purification and recycling of old powder to reduce cost, and the availability of new metal and alloy powders specifically designed for PBF. Also, there are a number of companies investing in larger-build stages to increase the output product size.
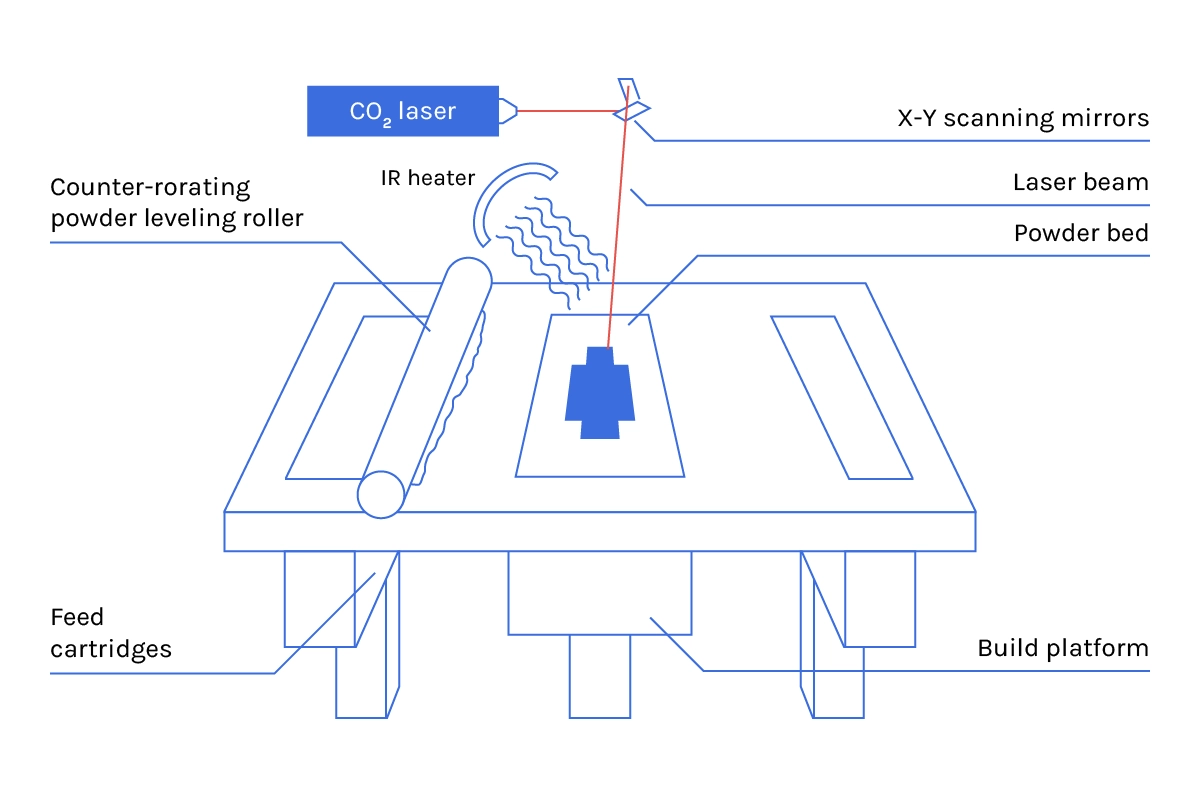
Source: Engineering Product Design
Direct energy deposition
Direct energy deposition (DED) builds parts by melting material as it is deposited using a focused thermal energy, such as a laser, electron beam or plasma arc. In the DED process, both the energy source head and the material feed nozzle are moved together on a gantry or robotic arm. DED is one of the faster AM processes used to produce high-strength, multi-material parts and to repair or add to existing parts. It is one of the lesser adopted processes in AM at the moment. Still, it has great potential as part of an integrated process with subtractive process or other AM processes because of its ability to add features and to layer on costings.
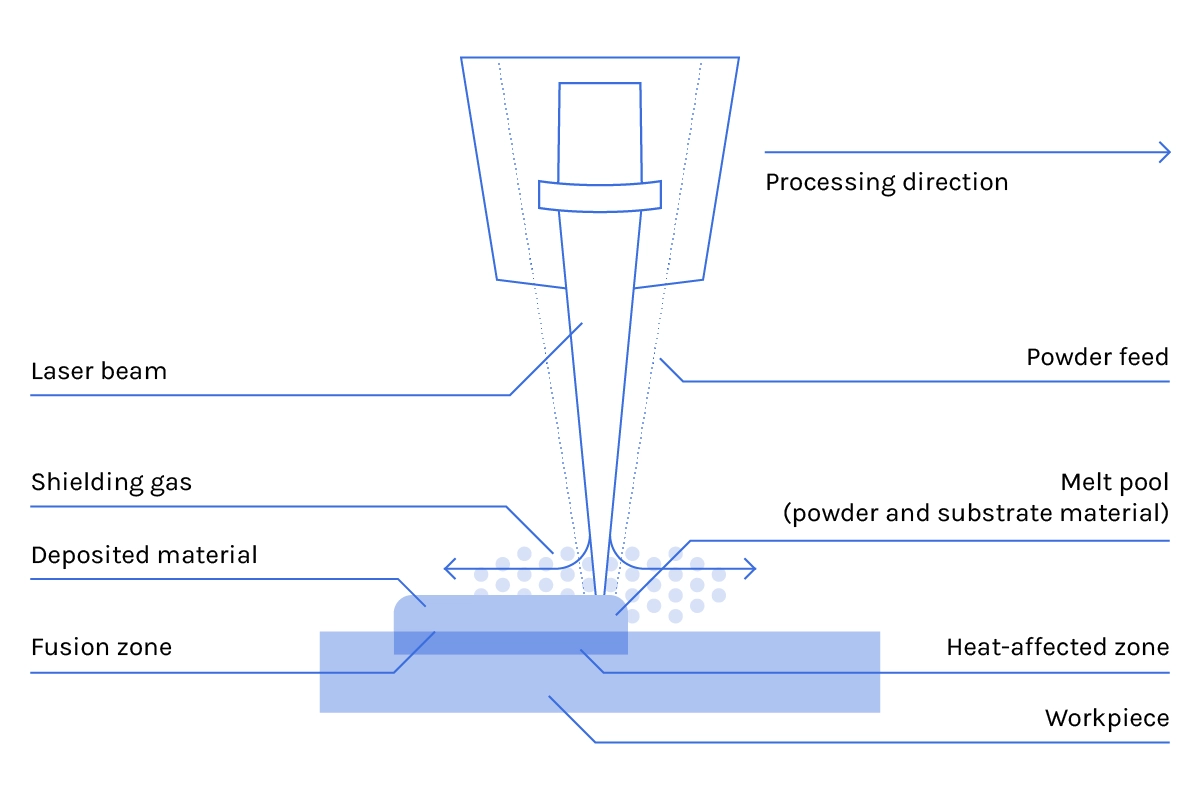
Source: Trumpf
Sheet lamination
Sheet lamination (SL) is the process of building an object by layering and laminating thin sheets of material and includes ultrasonic additive manufacturing (UAM) and laminated object manufacturing (LOM). The lamination method can be bonding, ultrasonic welding, or brazing while the final shape is achieved either by laser cutting or CNC machining.
The advantage of SL is the ability to achieve a composite of different material layers, improving sound insulation, strength, and other characteristics. It also requires minimal energy for this process. The accuracy of SL is one of its limiting functions, but because it is a faster and cheaper process, it is regularly used for prototyping and creating molds. With its simplicity, low cost, and growing material library, its uses continue to expand.
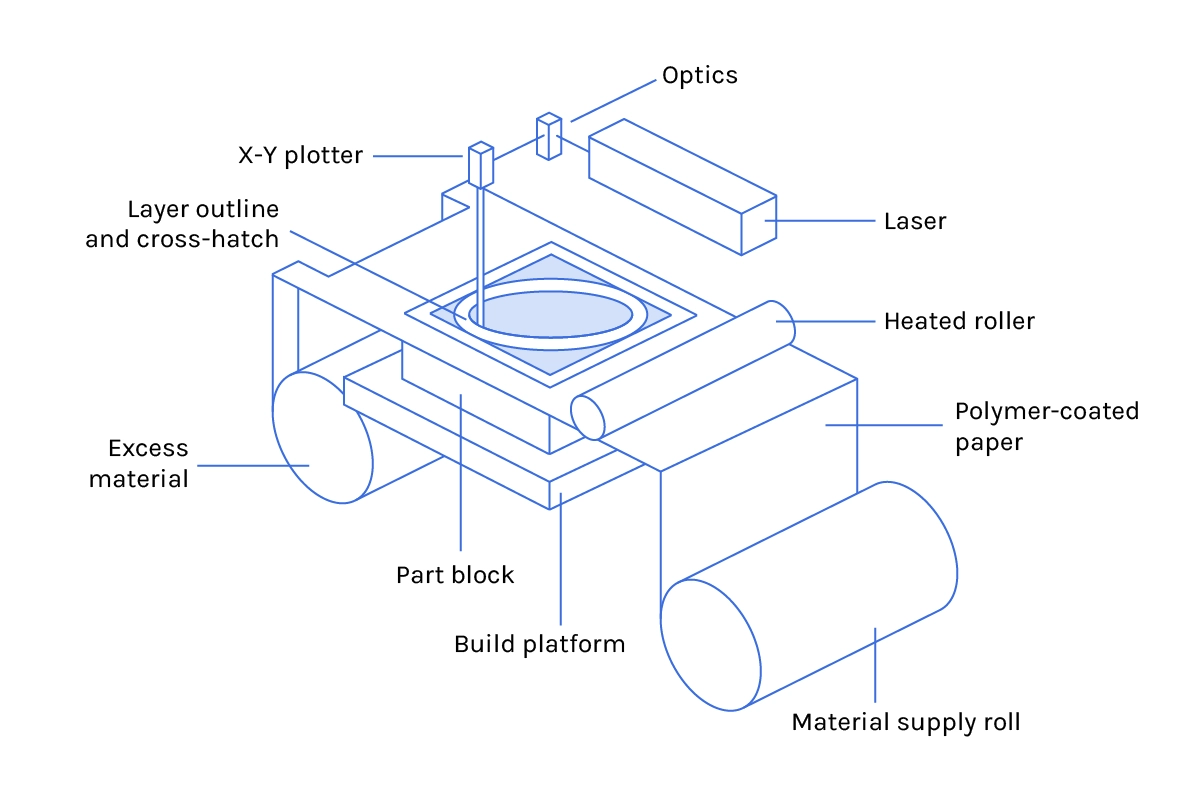
Source: Engineering Product Design
Fifth Layer: Post Processing
Once an AM printer has finished printing, there may still be some work to do on the final piece. For example, many shapes require supports to print properly. Removing those is a post-processing task. Some parts need to be sanded or given put a finish layer onto a piece. Resin-based prints, as opposed to filament, requirebased prints need to have any uncured resin to be washed off before and final curing under UV light. In the early days of AM, these costs, including depowdering, support removal, surface treatment, surface sealing, part identification, transportation, sorting, and packaging were often underestimated.
AM post processing is used to enhance the surface properties of prints in many aspects to deliver improved mechanical performance and aesthetic appearance. By improving these key surface characteristics, post processing widely extends the range of use cases and applications across all industries. For example, three types of post-processing techniques can be applied to fused filament fabrication (FFF) prints:
- Material Removal
- Material Addition
- Material Property Change
The continued advancements in the downstream AM post processing, including automation and inspection, and techniques to reduce damage during these processes are important to increasing efficiency and reducing costs.